8 Chapter 8: Meiosis and Sexual Reproduction: Meiosis II
Lisa Limeri; Joshua Reid; rocksher; and Shifath Bin Syed
Learning Objectives
By the end of this section, you will be able to do the following:
- Compare the process of mitosis with the process of meiosis II.
- Construct a model of meiosis (I and II) to demonstrate your understanding of the process.
- Describe how meiosis leads to the formation of gametes (egg or sperm).
Introduction
Recall from last chapter that:
- Meiosis is the process through which gametes are produced, with half the ploidy of the original cell (in most animals, somatic cells are diploid, and gametes are haploid)
- Meiosis involves two sets of cell division, which are called Meiosis I and Meiosis II
- In Meiosis I, homologous chromosomes separate, resulting in two haploid cells.
Meiosis II
In this chapter, we will explore Meiosis II in detail, in which sister chromatids separate, resulting in four haploid cells. In some species, cells enter brief interphase before entering meiosis II. This interphase lacks an S phase, so chromosomes are not duplicated. The two cells produced in meiosis I go through the events of meiosis II in synchrony. During meiosis II, the sister chromatids within the two daughter cells separate, forming four new haploid gametes. The mechanics of meiosis II are similar to those in mitosis, except that each dividing cell has only one set of homologous chromosomes, each with two chromatids. Therefore, each cell has half the number of sister chromatids to separate as a diploid cell undergoing mitosis.
Prophase II
If the chromosomes decondense in telophase I, they condense again. If nuclear envelopes were formed, they fragment into vesicles. The MTOCs duplicated during the brief interphase move away from each other toward opposite poles, and new spindles are formed.
Prometaphase II
The nuclear envelopes are completely broken down, and the spindle is fully formed. Each sister chromatid forms an individual kinetochore that attaches to microtubules at opposite poles.
Metaphase II
The sister chromatids are maximally condensed and aligned at the cell’s equator.
Anaphase II
The sister chromatids are pulled apart by the kinetochore microtubules and move toward opposite poles (Figure 8.1). Non-kinetochore microtubules elongate the cell.
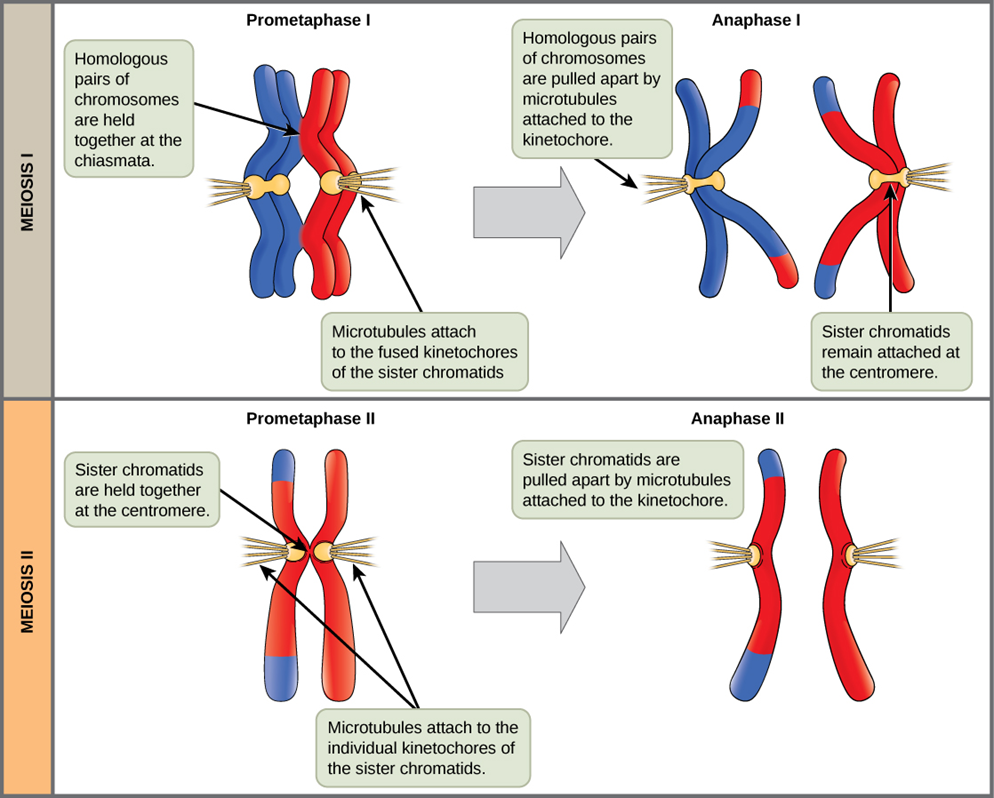
Telophase II and Cytokinesis
The chromosomes arrive at opposite poles and begin to decondense. Nuclear envelopes form around the chromosomes. If the original parent cell was diploid, as is most commonly the case, cytokinesis now separates the two into four unique haploid cells. The cells produced are genetically unique because of the random assortment of paternal and maternal homologous chromosomes and the recombination of maternal and paternal segments of chromosomes that occurs during crossover. The entire process of meiosis is outlined in Figure 8.2
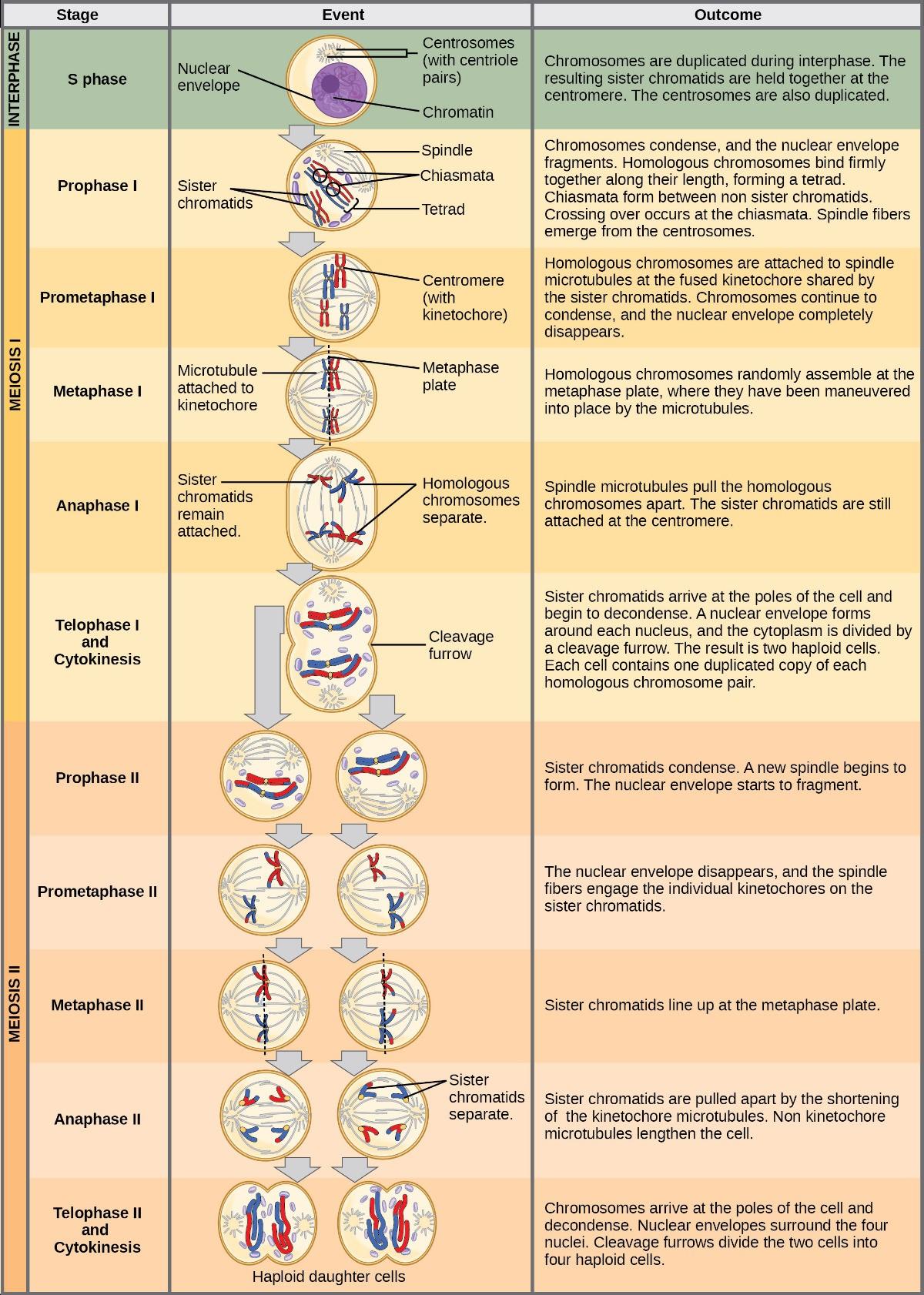
Reading Question #1
During which phase of meiosis II do sister chromatids separate and move toward opposite poles of the cell?
A. Prophase II
B. Prometaphase II
C. Metaphase II
D. Anaphase II
Reading Question #2
How many haploid cells are formed at the end of meiosis II if the starting cell is diploid?
A. 1 haploid cell
B. 2 haploid cells
C. 3 haploid cells
D. 4 haploid cells
Comparing Meiosis and Mitosis
Mitosis and meiosis are both forms of nuclear division in eukaryotic cells. They share some similarities but exhibit significant differences that lead to very different outcomes (Fig 8.4). Mitosis is a single nuclear division resulting in two nuclei, usually partitioned into two new cells. The nuclei resulting from a mitotic division are genetically identical to the original nucleus. They have the same number of chromosomes as each other and the parent cell: one set in the case of haploid cells and two sets in the case of diploid cells.
In contrast, meiosis consists of two nuclear divisions resulting in four nuclei that are usually partitioned into four new, genetically distinct cells. The four nuclei produced during meiosis are not genetically identical and contain half the number of chromosome sets as the original cell.
A stark difference between mitosis and meiosis occurs in meiosis I, which is a very different nuclear division from mitosis. In meiosis I, the homologous chromosome pairs physically meet and are bound to each other in the synaptonemal complex. Following this, the chromosomes develop chiasmata and undergo crossover between non-sister chromatids. In the end, the chromosomes line up along the metaphase plate as tetrads, with kinetochore fibers from opposite spindle poles attached to each kinetochore of a homolog to form a tetrad. When the chiasmata dissolve, and the tetrad is broken up, the homologs move towards opposite poles. When the homologous chromosomes (homologs) separate, the ploidy level (i.e., the number of sets of chromosomes in each future nucleus) reduces (typically from two to one). For this reason, meiosis I is referred to as a reductional division. All of these events occur only in meiosis I. There is no reduction in ploidy level during mitosis.
Meiosis II is analogous to a mitotic division. In this case, the duplicated chromosomes (only one set of them) line up on the metaphase plate with divided kinetochores attached to kinetochore fibers from opposite poles. During anaphase II, as in mitotic anaphase, the kinetochores divide, and one sister chromatid—now referred to as a chromosome—is pulled to one pole while the other sister chromatid is pulled to the other pole. If it were not for the fact that there had been a crossover, the two products of each individual meiosis II division would be identical (as in mitosis). Instead, they are different because there has always been at least one crossover per chromosome. Meiosis II is not a reduction division because, although there are fewer copies of the genome in the resulting cells, there is still one set of chromosomes, as there was at the end of meiosis I.
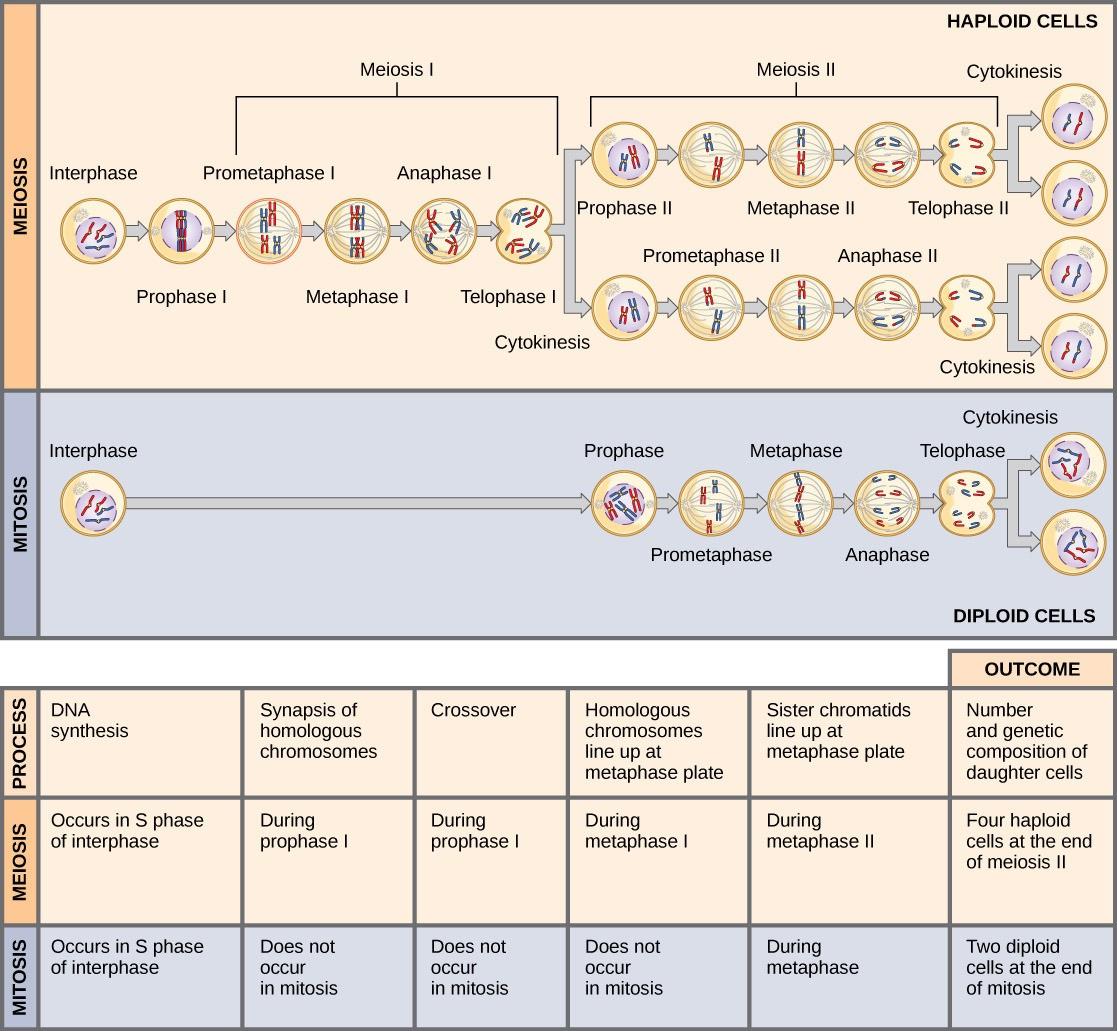
Evolution Connection: The Mystery of the Evolution of Meiosis
Some characteristics of organisms are so widespread and fundamental that it is sometimes difficult to remember that they evolved like other simple traits. Meiosis is such an extraordinarily complex series of cellular events that biologists have had trouble testing hypotheses about how it may have evolved. Although meiosis is inextricably entwined with sexual reproduction and its advantages and disadvantages, it is important to separate the questions of the evolution of meiosis and the evolution of sex because early meiosis may have been advantageous for different reasons than it is now. Thinking outside the box and imagining the early benefits of meiosis might have been one approach to uncovering how it may have evolved.
Meiosis and mitosis share evident cellular processes, and it makes sense that meiosis evolved from mitosis.
The difficulty lies in the clear differences between meiosis I and mitosis. Adam Wilkins and Robin Holliday (2009) summarized the unique events needed to evolve meiosis from mitosis. These steps are homologous chromosome pairing and synapsis, crossover exchanges, sister chromatids remaining attached during anaphase, and suppression of DNA replication in the interphase between meiosis I and meiosis II. They argue that the first step is the hardest and most important and that understanding how it evolved would clarify the evolutionary process. They suggest genetic experiments that might shed light on the evolution of synapsis.
There are other approaches to understanding the evolution of meiosis in progress. Different forms of meiosis exist in single-celled protists. Some appear to be simpler or more “primitive” forms of meiosis. Comparing the meiotic divisions of different protists may shed light on the evolution of meiosis. Marilee Ramesh and colleagues (2005) compared the genes involved in meiosis in protists to understand when and where meiosis might have evolved. Although research is ongoing, recent scholarship into meiosis in protists suggests that some aspects of meiosis may have evolved later. This kind of genetic comparison can tell us what aspects of meiosis are the oldest and what cellular processes they may have borrowed from in earlier cells.
Reading Question #3
What is the main difference between mitosis and meiosis in terms of the resulting cells?
A. Mitosis results in genetically identical cells, while meiosis results in genetically distinct cells.
B. Mitosis results in haploid cells, while meiosis results in diploid cells.
C. Mitosis results in four nuclei, while meiosis results in two nuclei.
D. Mitosis results in reduced ploidy, while meiosis results in increased ploidy.
Reading Question #4
Which event occurs during meiosis I but not during mitosis?
A. Separation of sister chromatids
B. Alignment of chromosomes at the metaphase plate
C. Synapsis and crossing over between homologous chromosomes
D. Formation of kinetochores and attachment to microtubules
Life Cycles of Sexually Reproducing Organisms
Fertilization and meiosis alternate in sexual life cycles. What happens between these two events depends on the organism’s reproductive strategy. The process of meiosis reduces the chromosome number by half. Fertilization, the joining of two haploid gametes, restores the diploid condition. Some organisms have the most obvious multicellular diploid stage and only produce haploid reproductive cells. Animals, including humans, have this type of life cycle. Other organisms, such as fungi, have the most obvious multicellular haploid stage. Plants and some algae have an alternation of generations, in which they have multicellular diploid and haploid life stages that are apparent to different degrees depending on the group.
Nearly all animals employ a diploid-dominant life-cycle strategy in which the only haploid cells produced by the organism are the gametes. Early in the embryo’s development, specialized diploid cells, called germ cells, are produced within the gonads (such as the testes and ovaries). Germ cells are capable of mitosis to perpetuate the germ cell line and meiosis to produce haploid gametes. Once the haploid gametes are formed, they lose the ability to divide again. There is no multicellular haploid life stage. Fertilization occurs with the fusion of two gametes, usually from different individuals, restoring the diploid state (Figure 8.5).
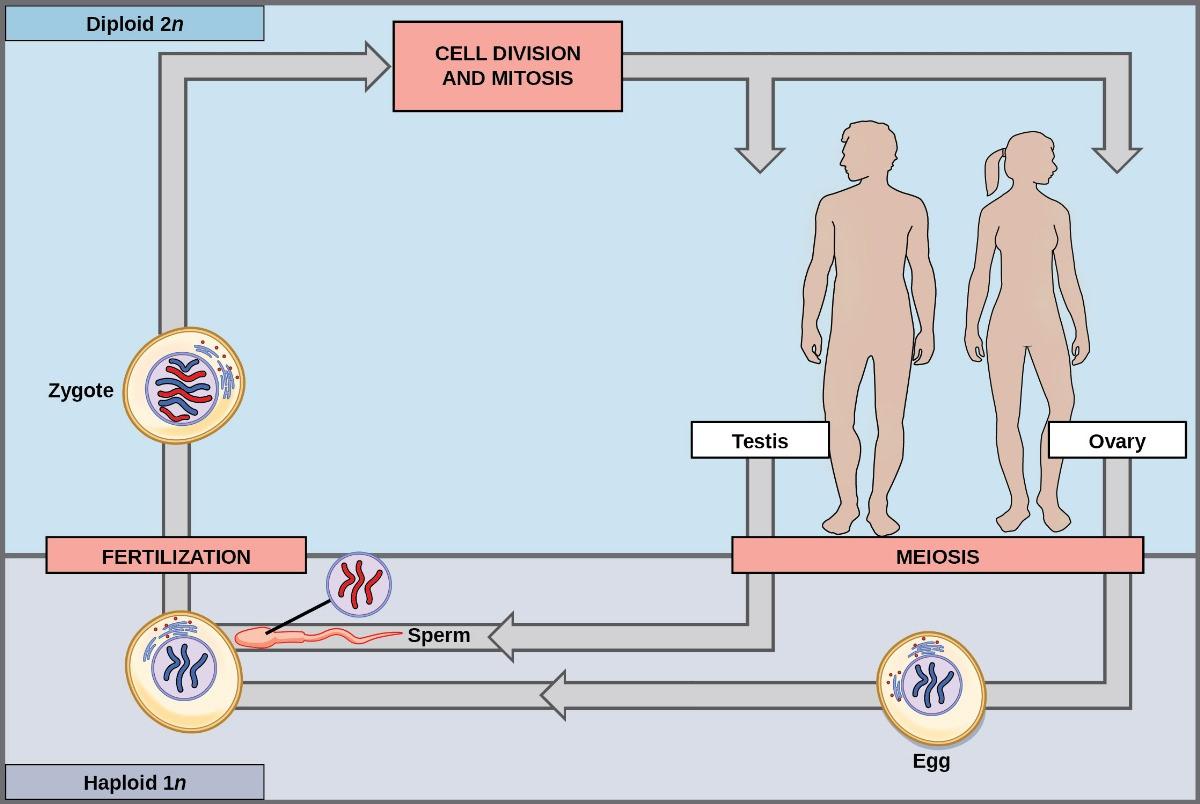
Most fungi and algae employ a life-cycle type in which the “body” of the organism—the ecologically important part of the life cycle—is haploid. The haploid cells that make up the tissues of the dominant multicellular stage are formed by mitosis. During sexual reproduction, specialized haploid cells from two individuals—designated the (+) and (−) mating types—join to form a diploid zygote. The zygote immediately undergoes meiosis to form four haploid cells called spores. Although these spores are haploid like the “parents,” they contain a new genetic combination from two parents. The spores can remain dormant for various periods. Eventually, when conditions are favorable, the spores form multicellular haploid structures through many rounds of mitosis (Figure 8.6).
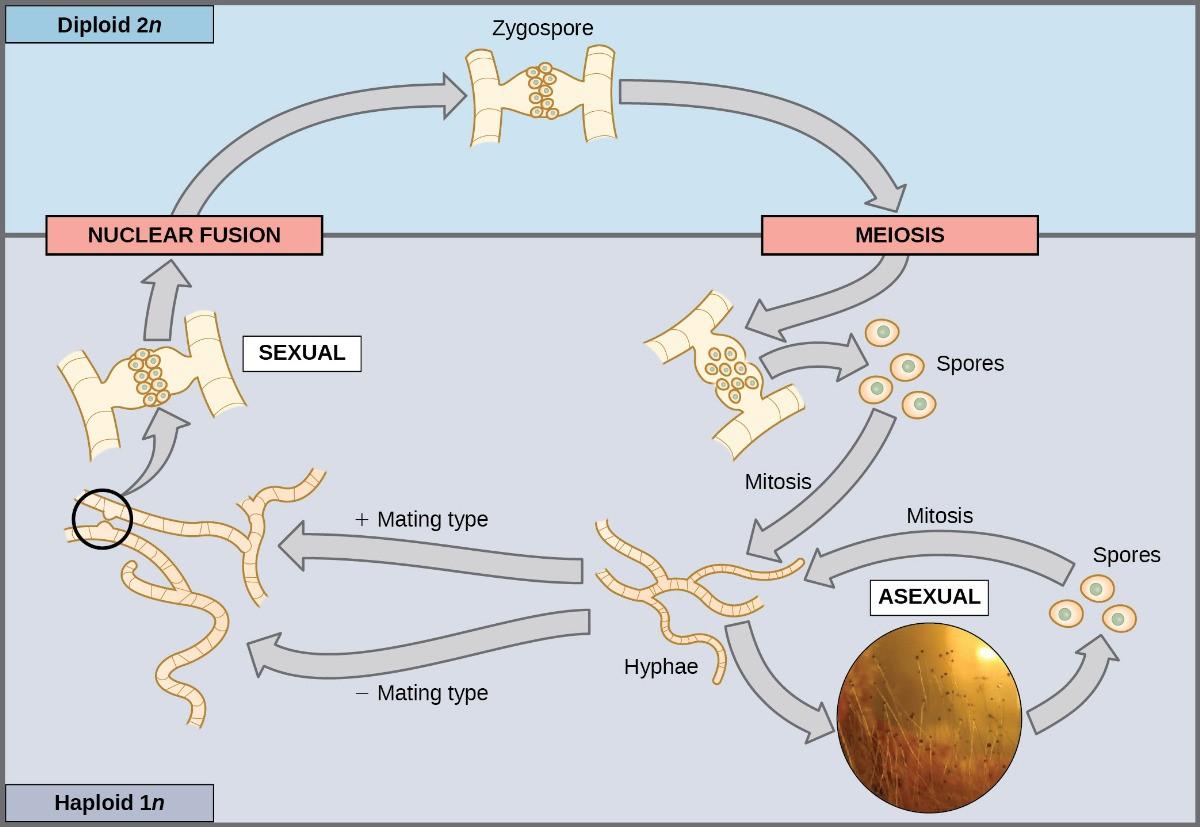
The third life-cycle type, employed by some algae and all plants, is a blend of the haploid-dominant and diploid-dominant extremes. Species with alternating generations have haploid and diploid multicellular organisms as part of their life cycle. The haploid, multicellular plants are called gametophytes because they produce gametes from specialized cells. Meiosis is not directly involved in the production of gametes because the organism that produces the gametes is already haploid. Fertilization between the gametes forms a diploid zygote. The zygote will undergo many rounds of mitosis and give rise to a diploid; multicellular plant called a sporophyte. Specialized cells of the sporophyte will undergo meiosis and produce haploid spores. The spores will subsequently develop into gametophytes (Figure 8.7).
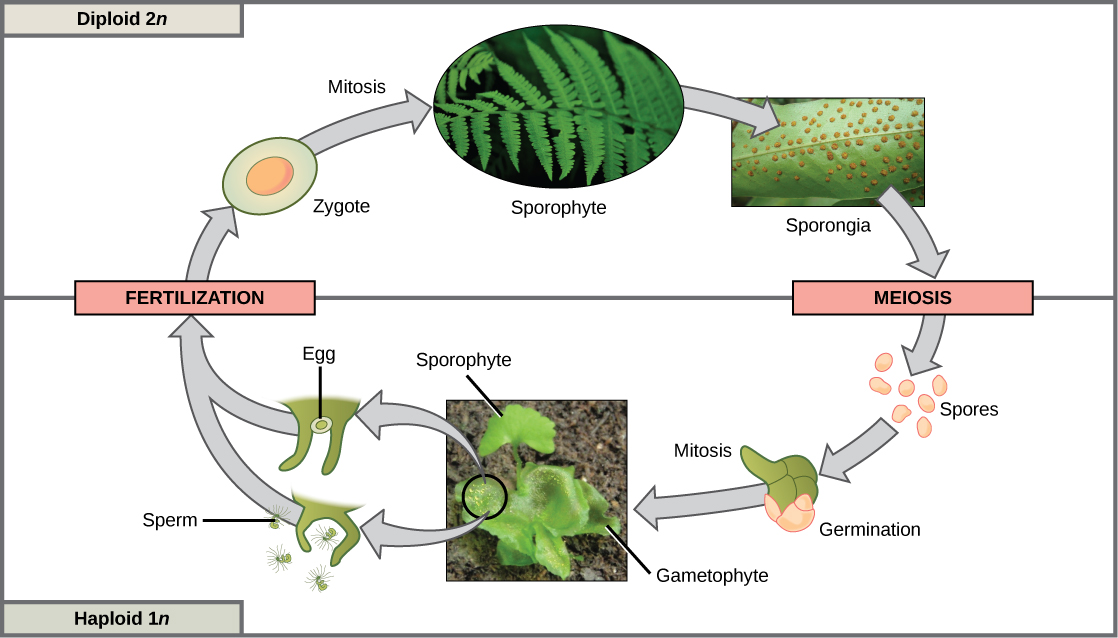
Although all plants utilize some version of the alternation of generations, the relative sizes of the sporophyte and the gametophyte and their relationship vary greatly. The gametophyte organism is free-living in plants such as moss, and the sporophyte is physically dependent on the gametophyte. In other plants, such as ferns, both the gametophyte and sporophyte plants are free-living; however, the sporophyte is much larger. In seed plants, such as magnolia trees and daisies, the gametophyte is composed of only a few cells and, in the case of the female gametophyte, is completely retained within the sporophyte.
Sexual reproduction takes many forms in multicellular organisms. The fact that nearly every multicellular organism on Earth employs sexual reproduction is strong evidence for the benefits of producing offspring with unique gene combinations. However, there are other possible benefits as well.
Reading Question #5
Which type of life cycle is characterized by a multicellular diploid stage and haploid reproductive cells?
A. Alternation of generations
B. Haploid-dominant life cycle
C. Diploid-dominant life cycle
D. Meiosis-dominant life cycle
Acknowledgements
Adapted from Clark, M.A., Douglas, M., and Choi, J. (2018). Biology 2e. OpenStax. Retrieved from https://openstax.org/books/biology-2e/pages/1-introduction