26 Chapter 26: Respiration: Glycolysis
Lisa Limeri; Joshua Reid; and rocksher
Learning Objectives
By the end of this section, you will be able to
- When given the metabolism pathways for the steps of respiration, identify redox reactions, including which atoms are being oxidized/reduced, and explain what this indicates about electron flow.
- Describe the energy flow in glycolysis, including identifying when energy is invested in the carbohydrate or released and the usable form in which it is captured (i.e., ATP or as NADH and FADH2).
- Explain how glycolysis is regulated, including identifying the regulatory steps and their regulatory mechanism.
Introduction
Virtually every task performed by living organisms requires energy. Organisms require energy to perform heavy labor and exercise, but humans also use considerable energy while thinking, and even during sleep. Every organism’s living cells constantly use energy. Cells break down complex carbohydrates into simple sugars that they use for energy.
A huge variety of cellular processes require a steady supply of energy. From where, and in what form, does this energy come? How do living cells obtain energy, and how do they use it? In this unit, we will explore, in depth, the process through which cells oxidize the carbons in carbohydrates and lipids to extract energy, called aerobic cellular respiration. In this module, we will begin by first overviewing different types of reactions that we will see repeatedly, and then explore the first stage of cellular respiration, glycolysis.
Energy and Metabolism
Cellular processes, such as building and breaking down complex molecules, occur through stepwise chemical reactions. Some of these chemical reactions are spontaneous and release energy; whereas, others require energy to proceed. Just as living things must continually consume food to replenish what they have used, cells must continually obtain more energy to replenish that which the many energy-requiring chemical reactions that constantly take place use (Fig 26.1). All of the chemical reactions that transpire inside cells, including those that use and release energy, are the cell’s metabolism.
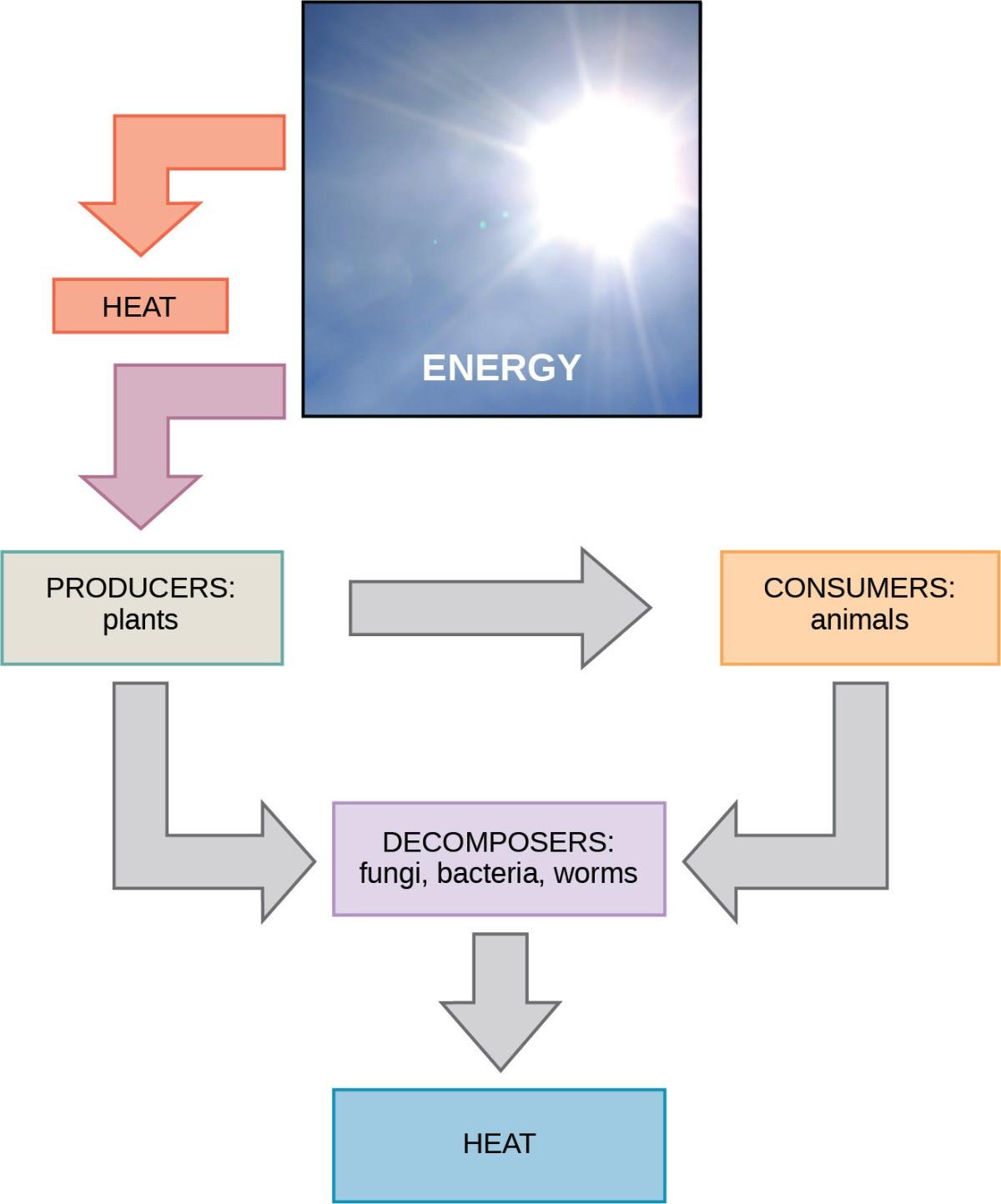
Carbohydrate Metabolism
Consumers consume glucose (a simple carbohydrate) as a major energy source, because glucose molecules have considerable energy stored within their bonds. The following equation describes the breakdown of glucose:
C6H12O6 + 6O2 → 6CO2 + 6H2O + energy
A single glucose molecule can store enough energy to make a great deal of ATP (~36 molecules) through a catabolic pathway called aerobic cellular respiration. Catabolic pathways involve degrading (or breaking down) complex molecules into simpler ones. Molecular energy stored in high-energy molecules are released in catabolic pathways and harvested in such a way that it can produce ATP. Other energy-storing molecules, such as fats, also break down through similar catabolic reactions to release energy and make ATP (Figure 26.2). The process of using oxygen to catabolize glucose and produce large amounts of ATP may be broken down into three major stages: glycolysis, pyruvate oxidation and the citric acid cycle, and oxidative phosphorylation. In this section, we will discuss the first major stage, glycolysis.

Reading check #1
What is the primary purpose of catabolic pathways in cellular metabolism?
A. To build complex molecules from simpler ones
B. To store energy in chemical bonds
C. To capture sunlight for energy
D. To break down complex molecules into simpler ones and release energy
Enzymes Catalyze Reactions
All chemical reactions require an input of energy to begin, called activation energy. This is because it always takes an initial input of energy to break a bond, even if after the bond is broken, the products are at a lower energy state than the reactants. To break bonds, the atoms must be contorted, which is called the transition state, and it is a high-energy, unstable state. For this reason, reactant molecules do not last long in their transition state, but very quickly proceed to the chemical reaction’s next steps.
A substance that helps a chemical reaction to occur is a catalyst, and the special molecules that catalyze biochemical reactions are enzymes. Almost all enzymes are proteins. Enzymes lower the activation energy by binding to the reactant molecules, and holding them in such a way as to make the chemical bond-breaking and bond-forming processes take place more readily.
Reading Check #2
Which term describes the initial input of energy required to break a chemical bond and initiate a reaction?
A. Enzyme activity
B. Substrate specificity
C. Activation energy
D. Transition state
Enzyme Active Site and Substrate Specificity
The chemical reactants to which an enzyme binds are the enzyme’s substrates. There may be one or more substrates, depending on the particular chemical reaction. The location within the enzyme where the substrate binds is the enzyme’s active site. This is where the “action” happens. In protein enzymes, there is a unique combination of amino acid side chains within the active site. Different properties characterize each side chain. These can be large or small, acidic or basic, hydrophilic or hydrophobic, positively or negatively charged, or neutral. The unique combination of amino acid residues, their positions, sequences, structures, and properties, creates a very specific chemical environment within the active site. This specific environment is suited to bind, albeit briefly, to a specific chemical substrate (or substrates). Due to this jigsaw puzzle-like match between an enzyme and its substrates, enzymes are known for their specificity. The “best fit” results from the shape and the amino acid functional group’s attraction to the substrate. There is a specifically matched enzyme for each substrate and, thus, for each chemical reaction.
Induced Fit and Enzyme Function
For many years, scientists thought that enzyme-substrate binding took place in a simple “lock-and-key” fashion. This model asserted that the enzyme and substrate fit together perfectly in one instantaneous step. However, current research supports a more refined view scientists call induced fit (Figure 26.3). This model expands upon the lock-and-key model by describing a more dynamic interaction between enzyme and substrate. As the enzyme and substrate come together, their interaction causes a mild shift in the enzyme’s structure that confirms an ideal binding arrangement between the enzyme and the substrate’s transition state. This ideal binding maximizes the enzyme’s ability to catalyze its reaction.
When an enzyme binds its substrate, it forms an enzyme-substrate complex. This complex lowers the reaction’s activation energy and promotes its rapid progression in one of many ways. On a basic level, enzymes promote chemical reactions that involve more than one substrate by bringing the substrates together in an optimal orientation. The appropriate region (atoms and bonds) of one molecule is juxtaposed to the other molecule’s appropriate region with which it must react. Another way in which enzymes promote substrate reaction is by creating an optimal environment within the active site for the reaction to occur. Certain chemical reactions might proceed best in a slightly acidic or non-polar environment. The chemical properties that emerge from the particular arrangement of amino acid residues within an active site create the perfect environment for an enzyme’s specific substrates to react. After an enzyme catalyzes a reaction, it releases its product(s).
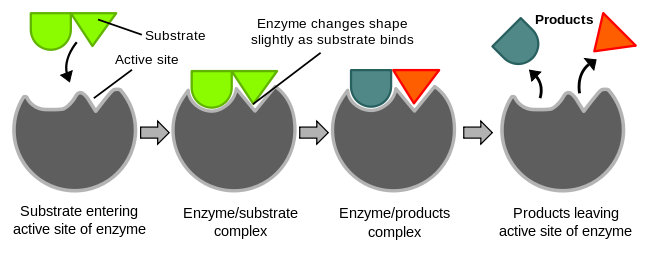
Energy stored in usable form as ATP in Living Systems
A living cell cannot store significant amounts of free energy. Excess free energy would result in an increase of heat in the cell, which would result in excessive thermal motion that could damage the cell. Rather, a cell must be able to handle that energy in a way that enables the cell to store energy safely and release it for use only as needed. Living cells accomplish this by using the compound adenosine triphosphate (ATP). ATP is often called the “energy currency” of the cell, and, like currency, this versatile compound can be used to fill any energy need of the cell. How? It functions similarly to a rechargeable battery.
When ATP is broken down by the removal of its terminal phosphate group, energy is released. The energy is used to do work by the cell, usually when the released phosphate binds to another molecule, thereby activating it. A reaction that adds a phosphate group to a molecule is called a phosphorylation reaction; a reaction that removes a phosphate from a molecule is called a dephosphorylation reaction.
Kinase is the name given to the group of enzymes that catalyze phosphorylation reactions. For example, in the mechanical work of muscle contraction, ATP supplies the energy to move the contractile muscle proteins. Recall the active transport work of the sodium-potassium pump in cell membranes. ATP alters the structure of the integral protein that functions as the pump, changing its affinity for sodium and potassium. In this way, the cell performs work, pumping ions against their electrochemical gradients.
ATP Structure and Function
At the heart of ATP is a molecule of adenosine monophosphate (AMP), which is composed of an adenine nitrogenous base bonded to a ribose molecule and to a single phosphate group (Figure 26.4). AMP is one of the nucleotides in RNA. The addition of a second phosphate group to this core molecule results in the formation of adenosine diphosphate (ADP); the addition of a third phosphate group forms adenosine triphosphate (ATP). Phosphate groups are negatively charged and thus repel one another when they are arranged in series, as they are in ADP and ATP. This repulsion makes the ADP and ATP molecules inherently unstable. The release of one or two phosphate groups from ATP, a process called dephosphorylation, releases energy.
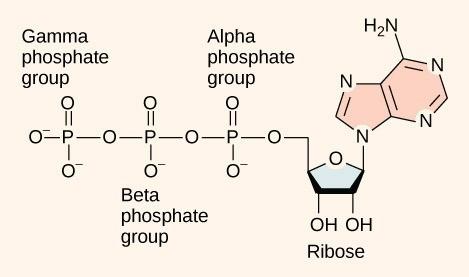
Energy from ATP
Hydrolysis is the process of using a water molecule to break complex macromolecules apart (hydro = water; lysis = to break apart). During hydrolysis, water is split, or lysed, and the resulting hydrogen atom (H+) and a hydroxyl group (OH–), or hydroxide, are added to the larger molecule. The hydrolysis of ATP produces ADP, an inorganic phosphate ion (Pi), and the release of free energy. To carry out life processes, ATP is continuously broken down into ADP, and like a rechargeable battery, ADP is continuously regenerated into ATP by the reattachment of a third phosphate group. Water, which was broken down into its hydrogen atom and hydroxyl group (hydroxide) during ATP hydrolysis, is regenerated when a third phosphate is added to the ADP molecule, reforming ATP.
Obviously, energy must be infused into the system to regenerate ATP. Where does this energy come from? In nearly every living thing on Earth, the energy comes from the metabolism of carbohydrates, most commonly glucose. In this way, ATP is a direct link between the limited set of exergonic pathways of glucose catabolism and the multitude of endergonic pathways that power living cells.
Glycolysis
Glycolysis is the first step in the breakdown of glucose to extract energy for cellular metabolism. In fact, nearly all living organisms carry out glycolysis as part of their metabolism. Glycolysis takes place in the cytoplasm of both prokaryotic and eukaryotic cells.
Glycolysis begins with the six-carbon glucose molecule and ends with two molecules of a three-carbon carbohydrate called pyruvate. Glycolysis consists of two distinct phases. The first part of the glycolysis pathway, called the energy investment phase, traps the glucose molecule in the cell and uses energy to modify it so that the six-carbon sugar molecule can be split evenly into the two three-carbon molecules. The second part of glycolysis, called the energy harvesting phase, extracts energy from the molecules and stores it in the form of ATP and NADH (the reduced form of NAD+).
First Half of Glycolysis (Energy Investment Phase)
Step 1: The first step in glycolysis (Figure 26.5) is catalyzed by hexokinase, an enzyme that catalyzes the phosphorylation of six-carbon sugars. Based on the name alone, you could infer that hexokinase would add a phosphate group to a molecule, because kinases catalyze phosphorylation reactions. Hexokinase phosphorylates glucose using ATP as the source of the phosphate, producing glucose-6-phosphate (G6P), a higher-energy and thus more reactive molecule. This reaction prevents the phosphorylated glucose molecule from leaving the cell because it is now negatively charged and can no longer move through glucose transport proteins on the cell’s membrane. This traps the glucose molecule inside the cell.
Step 2: In the second step of glycolysis, an isomerase converts glucose-6-phosphate into one of its isomers, fructose-6-phosphate (this isomer has a phosphate attached at the location of the sixth carbon). An isomerase is an enzyme that catalyzes the conversion of a molecule into one of its isomers, called an isomerization reaction. It is necessary to convert phosphoglucose to phosphofructose in order to later split of the sugar into two three-carbon molecules.
Step 3: The third step is the phosphorylation of fructose-6-phosphate, catalyzed by the enzyme phosphofructokinase. A second ATP molecule donates a high-energy phosphate to fructose-6-phosphate, producing fructose-1,6-bisphosphate (F1,6BP). In this pathway, phosphofructokinase is a rate-limiting enzyme. It is active when the concentration of ADP is high; it is less active when ADP levels are low and the concentration of ATP is high. Thus, if there is sufficient ATP in the system, the pathway slows down. This is a type of end product inhibition, since ATP is the end product of glucose catabolism. This is an elegant system to control metabolism in the cell. If ample ATP is available, there is no need to metabolize more glucose, which may end up being wasteful.
Step 4: The newly added high-energy phosphates further destabilize fructose-1,6-bisphosphate. The fourth step in glycolysis employs an enzyme, aldolase, to cleave fructose-1,6-bisphosphate into two three-carbon isomers: dihydroxyacetone phosphate and glyceraldehyde-3-phosphate.
Step 5: In the fifth step, an isomerase transforms the dihydroxyacetone-phosphate into its isomer, glyceraldehyde-3-phosphate. Thus, the pathway will continue with two molecules of a glyceraldehyde-3phosphate.
At this point in the pathway, there has been a net investment of energy from two ATP molecules in the breakdown of one glucose molecule.
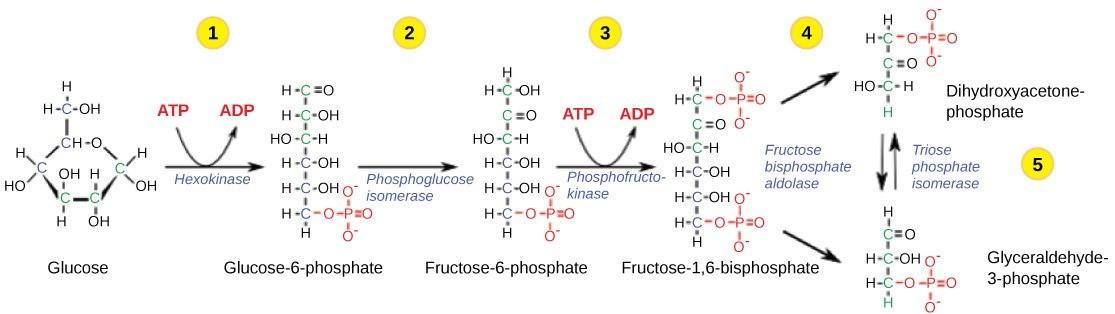
Second Half of Glycolysis (Energy Harvesting Phase)
So far, glycolysis has cost the cell two ATP molecules and produced two small, three-carbon sugar molecules. Both of these molecules will proceed through the second half of the pathway, and sufficient energy will be extracted to pay back the two ATP molecules used as an initial investment and produce a profit for the cell of two additional ATP molecules and two even higher-energy NADH molecules (Fig 26.6).
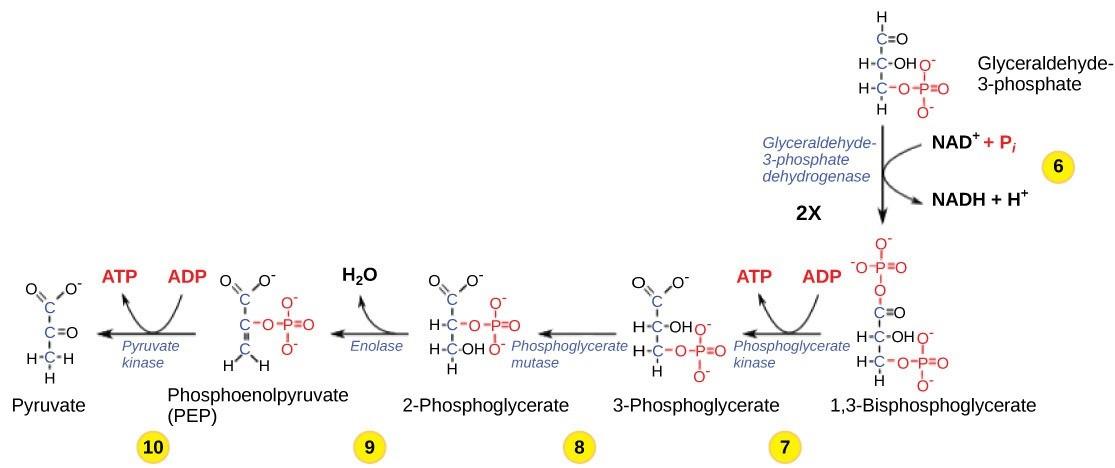
Step 6: The sixth step in glycolysis (Figure 26.6) oxidizes the sugar glyceraldehyde-3-phosphate, extracting high-energy electrons, which are picked up by the electron carrier NAD+, producing NADH. Since this happens twice (once for each glyceraldehyde-3-phosphate molecule), this results in the production of 2 NADH molecules per original glucose. Each glyceraldehyde-3-phosphate is then phosphorylated by the addition of a second phosphate group, producing 1,3-bisphosphoglycerate.
Here again is a potential limiting factor for this pathway. The continuation of the reaction depends upon the availability of the oxidized form of the electron carrier, NAD+. Thus, NADH must be continuously oxidized back into NAD+ in order to keep this step going. If NAD+ is not available, the second half of glycolysis slows down or stops. If oxygen is available in the system, the NADH will be oxidized readily and the high-energy electrons from the hydrogen released in this process will be used to produce ATP (oxidative phosphorylation, the last step of respiration). In an environment without oxygen, an alternate pathway (fermentation) can provide the oxidation of NADH to NAD+.
Step 7: In the seventh step, catalyzed by phosphoglycerate kinase, 1,3-bisphosphoglycerate donates a high-energy phosphate to ADP, forming one molecule of ATP. This is an example of substrate-level phosphorylation. This happens for each molecule of 1,3-bisphosphoglycerate, so two ATP molecules are produced per glucose at this step. A carbonyl group on the 1,3 bisphosphoglycerate is oxidized to a carboxyl group, and 3-phosphoglycerate is formed.
Step 8: In the eighth step, the remaining phosphate group in 3-phosphoglycerate moves from the third carbon to the second carbon, producing 2-phosphoglycerate (an isomer of 3-phosphoglycerate). The enzyme catalyzing this step is a mutase, which is an isomerase.
Step 9: Enolase catalyzes the ninth step. This enzyme causes 2-phosphoglycerate to lose water from its structure; this is a dehydration reaction, resulting in the formation of a double bond that increases the potential energy in the remaining phosphate bond and produces phosphoenolpyruvate (PEP).
Step 10: The last step in glycolysis is catalyzed by the enzyme pyruvate kinase (the enzyme in this case is named for the reverse reaction of pyruvate’s conversion into PEP) and results in the production of a second ATP molecule by substrate-level phosphorylation and pyruvate. Many enzymes in enzymatic pathways are named for the reverse reactions, since the enzyme can catalyze both forward and reverse reactions (these may have been described initially by the reverse reaction that takes place in vitro, under non physiological conditions).
Reading Check #5
What is the primary outcome of glycolysis?
A. Production of glucose
B. Production of two pyruvate molecules, ATP, and NADH
C. Synthesis of ATP
D. Formation of glucose-6-phosphate
Substrate Phosphorylation of ATP during glycolysis
ATP is generated through two mechanisms during the breakdown of glucose. A few ATP molecules are generated (that is, regenerated from ADP) as a direct result of the chemical reactions that occur in the catabolic pathways. A phosphate group is removed from an intermediate reactant in the pathway, and the free energy of the reaction is used to add the third phosphate to an available ADP molecule, producing ATP (Figure 26.7). This very direct method of phosphorylation is called substrate-level phosphorylation. This is in contrast with chemiosmosis, which uses secondary active transport to phosphorylate ADP to create ATP. Revisit the light-dependent reactions of photosynthesis module if you need a referesher on chemiosmosis. You will see this same mechanism re-appear later in aerobic respiration as the mechanism through which most of the ATP from respiration is produced.
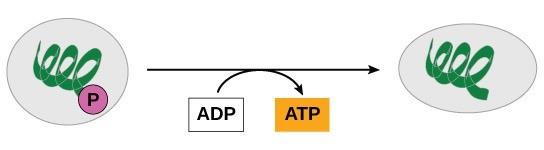
Reading Check #4
What type of reaction releases energy from ATP by removing a phosphate group from the molecule?
A. Hydrolysis reaction
B. Substrate-level phosphorylation
C. Dephosphorylation reaction
D. Phosphorylation reaction
Outcomes of Glycolysis
Glycolysis begins with glucose and ultimately produces two pyruvate molecules, net two ATP molecules, and two NADH molecules. (Note: two ATP molecules are used in the first half of the pathway to prepare the six glucose for cleavage, and produces four ATP molecules in the energy-harvesting phase, so the cell has a net gain of two ATP molecules). If the cell cannot catabolize the pyruvate molecules further, it will harvest only two ATP molecules from one molecule of glucose.
Metabolism Control Through Enzyme Regulation
Cellular needs and conditions vary from cell to cell, and change within individual cells over time. The required enzymes and energetic demands of stomach cells are different from those of fat storage cells, skin cells, blood cells, and nerve cells. Furthermore, a digestive cell works much harder to process and break down nutrients during the time that closely follows a meal compared with many hours after a meal. As these cellular demands and conditions vary, so do the amounts and functionality of different enzymes.
Since the rates of biochemical reactions are controlled by activation energy, and enzymes lower and determine activation energies for chemical reactions, it is enzymes that determine which reactions will proceed and at which rates. This determination is tightly controlled. In certain cellular environments, environmental factors like pH and temperature partly control enzyme activity. There are other mechanisms through which cells control enzyme activity and determine the rates at which various biochemical reactions will occur.
Molecular Regulation of Enzymes
Enzymes can be regulated in ways that either promote or reduce their activity. There are many different kinds of molecules that inhibit or promote enzyme function, and various mechanisms exist for doing so. For example, in some cases of enzyme inhibition, an inhibitor molecule is similar enough to a substrate that it can bind to the active site and simply block the substrate from binding. This is called competitive inhibition because an inhibitor molecule competes with the substrate for active site binding.
Some inhibitor molecules bind to enzymes in a location where their binding induces a conformational change that reduces the enzyme’s affinity for its substrate. This type of inhibition is an allosteric inhibition (also called noncompetitive inhibition) (Figure 26.8). More than one polypeptide comprise most allosterically regulated enzymes, meaning that they have more than one protein subunit. When an allosteric inhibitor binds to an enzyme, all active sites on the protein subunits change slightly such that they bind their substrates with less efficiency. There are allosteric activators as well as inhibitors. Allosteric activators bind to locations on an enzyme away from the active site, inducing a conformational change that increases the affinity of the enzyme’s active site(s) for its substrate(s).

Everyday Connection: Drug Discovery
Enzymes are key components of metabolic pathways. Understanding how enzymes work and how they can be regulated is a key principle behind developing many pharmaceutical drugs on the market today. Biologists working in this field collaborate with other scientists, usually chemists, to design drugs.
Consider statins for example—which is a class of drugs that reduces cholesterol levels. These compounds are essentially inhibitors of the enzyme HMG-CoA reductase, the enzyme that synthesizes cholesterol from lipids in the body. By inhibiting this enzyme, the drug reduces cholesterol levels synthesized in the body.
How are drugs developed? One of the first challenges in drug development is identifying the specific molecule that the drug is intended to target. Researchers identify targets through painstaking research in the laboratory. Identifying the target alone is not sufficient. Scientists also need to know how the target acts inside the cell and which reactions go awry in the case of disease. Once researchers identify the target and the pathway, then the actual drug design process begins. During this stage, chemists and biologists work together to design and synthesize molecules that can either block or activate a particular reaction. However, this is only the beginning: both if and when a drug prototype is successful in performing its function, then it must undergo many tests from in vitro experiments to clinical trials before it can obtain FDA approval to be on the market.
Negative Feedback Inhibition in Metabolic Pathways
Molecules can regulate enzyme function in many ways. Negative feedback inhibition a reaction product regulates its own further production (Figure 26.9). The cell responds to the abundance of specific products by slowing down further production of that molecule.
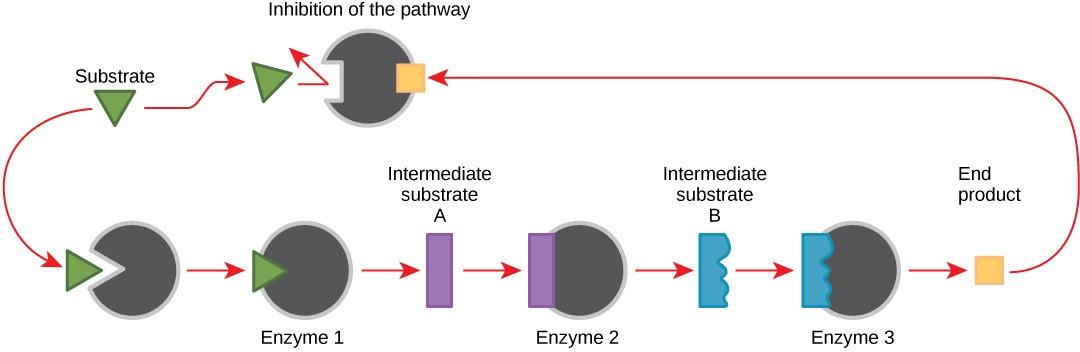
Producing both amino acids and nucleotides is controlled through feedback inhibition. Additionally, ATP is an allosteric regulator of some of the enzymes involved in sugar’s catabolic breakdown, the process that produces ATP. In this way, when ATP is abundant, the cell can prevent its further production. Remember that ATP is an unstable molecule that can spontaneously dissociate into ADP. If too much ATP were present in a cell, much of it would go to waste. Alternatively, ADP serves as a positive allosteric regulator (an allosteric activator) for some of the same enzymes that ATP inhibits. Thus, when relative ADP levels are high compared to ATP, the cell is triggered to produce more ATP through sugar catabolism.
Reading check #3
Which of the following is a key role of feedback inhibition in cellular metabolism?
A. Promoting the production of reaction products
B. Speeding up anabolic reactions
C. Regulating the production of specific molecules based on their abundance
D. Inhibiting all enzymatic reactions in a metabolic pathway
Regulation of Glycolysis
There are three major enzymatic control points within the glycolytic pathway: hexokinase, phosphofructokinase, and pyruvate kinase (Figure 26.10). There are multiple ways to elicit changes in the activity of these enzymes, including product inhibition, metabolites that act as allosteric modulators, signaling pathways that cause phosphorylation or acetylation of the enzymes, and changes in concentrations of these enzymes caused by transcriptional fluctuations. Key drivers for regulating the pathway are energy demand within the cell as determined by local indicators such as ATP and AMP, as well as energy demand within the organism as a whole, which can be influenced by hormone signaling pathways. The regulation of the pathway can vary depending on cell type and cellular needs.

Hexokinase Regulation
Recall that hexokinase enzymes mediate the first step in the glycolytic pathway with the formation of glucose 6-phosphate. Hexokinase is regulated via negative feedback inhibition. It is inhibited by its own product, Glucose-6-Phosphate (G6P). Essentially, you do not want to waste time and energy making more than you need. Step 1 requires ATP, so this step is regulated to prevent needlessly spending valuable ATP.
Phosphofructokinase Regulation
Recall that phosphofructokinase-1 (PFK1) mediates the third step in the glycolytic pathway with the conversion of fructose 6-phosphate (F6P) to fructose 1,6-bisphosphate (F1,6BP). This is the committed step within the pathway. The phosphorylation of F6P to F1,6BP commits the F1,6BP to continue through the glycolytic pathway. It cannot be utilized for any other purpose at that point. F6P, on the other hand, could be converted back into glucose-6-phosphate and used for many different purposes (ie glycogen synthesis, nucleotide synthesis, or hexosamine synthesis). Because of the committed nature of this step, PFK1 is one of the most important control points in the glycolytic pathway. PFK is regulated by multiple mechanisms (Figure 26.10). It is activated by the allosteric binding of ADP or AMP. High levels of ADP indicates a low energy state within the cell and the need for glycolysis and energy generation. Allosteric inhibitors include high levels of ATP and Citrate. Note that ATP is a substrate of this enzyme and has the normal substrate binding site. When there is enough ATP present that it can also bind allosterically to the enzyme, it will act as an inhibitor. Citrate, the first molecule in the Kreb’s Cycle (Citric Acid Cycle), can also act as an allosteric inhibitor of PFK. High levels of citrate indicate that no more pyruvate is needed to generate ATP through oxidative phosphorylation.
Pyruvate Kinase Regulation
Acknowledgements
Adapted from:
Clark, M.A., Douglas, M., and Choi, J. (2018). Biology 2e. OpenStax. Retrieved from https://openstax.org/books/biology-2e/pages/1-introduction
and
Jakubowski, H. & Flatt, P., (2023) Fundamentals of Biochemistry. LibreTexts. Retrieved from https://bio.libretexts.org/Bookshelves/Biochemistry/Fundamentals_of_Biochemistry_(Jakubowski_and_Flatt)
and
Lumen Learning. Microbiology. Retrieved from https://courses.lumenlearning.com/suny-microbiology/chapter/energy-matter-and-enzymes/