13 Chapter 13: Non-Mendelian Inheritance
Lisa Limeri; Joshua Reid; rocksher; and Shifath Bin Syed
Learning Objectives
By the end of this section, you will be able to do the following:
- Compare and contrast incomplete dominance, codominance, multiple alleles, polygenic, pleiotropy, epistasis, and epigenetics.
Introduction
Mendel’s experiments with pea plants suggested that: (1) two alleles exist for every gene; (2) alleles maintain their integrity in each generation (no blending); and (3) in the presence of the dominant allele, the recessive allele is hidden and makes no contribution to the phenotype. Therefore, recessive alleles can be “carried” and not expressed by individuals. Such heterozygous individuals are sometimes referred to as “carriers.” Genetic studies in other plants and animals have shown that while the Mendel’s principles are true for some traits, much more complexity exists. In this chapter, we consider some of the extensions of these Mendelian principles. If Mendel had chosen an experimental system that exhibited these genetic complexities, it’s possible that he would not have understood what his results meant.
Incomplete Dominance
Mendel’s finding that traits are inherited as dominant and recessive pairs contradicted the view that offspring exhibited a blend of their parent’s traits at that time. However, the heterozygote phenotype occasionally does appear to be intermediate between the two parents. For example, in the snapdragon, Antirrhinum majus (Figure 13.1), a cross between a homozygous parent with white flowers (CWCW) and a homozygous parent with red flowers (CRCR) will produce offspring with pink flowers (CRCW). (Note that different genotypic abbreviations are used for Mendelian extensions to distinguish these patterns from simple dominance and recessiveness.) This inheritance pattern is described as incomplete dominance, denoting the expression of two contrasting alleles such that the individual displays an intermediate phenotype. The allele for red flowers is incompletely dominant over the allele for white flowers. However, the results of a heterozygote self-cross can still be predicted, just as with Mendelian dominant and recessive crosses. In this case, the genotypic ratio would be 1 CRCR:2 CRCW:1 CWCW, and the phenotypic ratio would be 1:2:1 for red:pink:white.
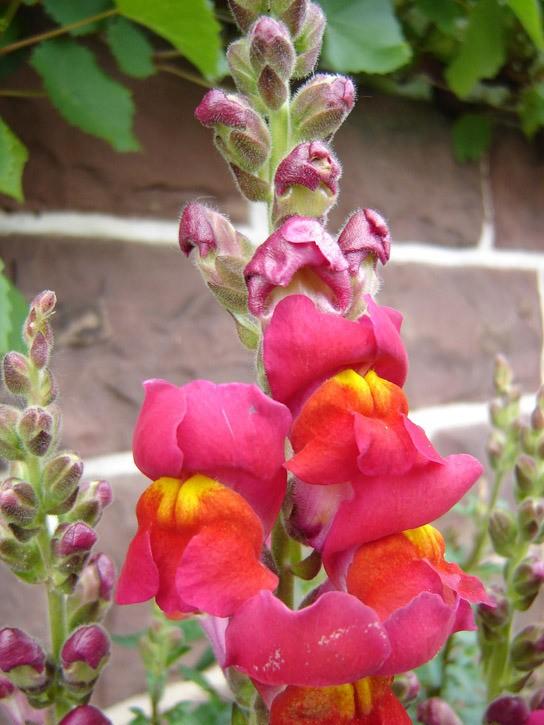
Reading Question #1
What is the term used to describe the phenomenon where one mutant allele is dominant over all other phenotypes, including the wild type?
A. Incomplete dominance
B. Codominance
C. Dominance hierarchy
D. Complete dominance
Reading Question #2
What is the characteristic of the heterozygote phenotype in incomplete dominance?
A. It shows a blend of the two parental traits.
B. It exhibits an intermediate phenotype between the two parental traits.
C. It expresses both alleles simultaneously.
D. It hides the recessive allele and only expresses the dominant allele.
Codominance
A variation on incomplete dominance is codominance, in which both alleles for the same characteristic are simultaneously expressed in the heterozygote. An example of codominance is the MN blood groups of humans. The M and N alleles are expressed in the form of an M or N antigen present on the surface of red blood cells. Homozygotes (LMLM and LNLN) express either the M or the N allele, and heterozygotes (LMLN) express both alleles equally. The three possible offspring genotypes are phenotypically distinct in a self-cross between heterozygotes expressing a codominant trait. However, a Mendelian monohybrid cross’s 1:2:1 genotypic ratio characteristic still applies.
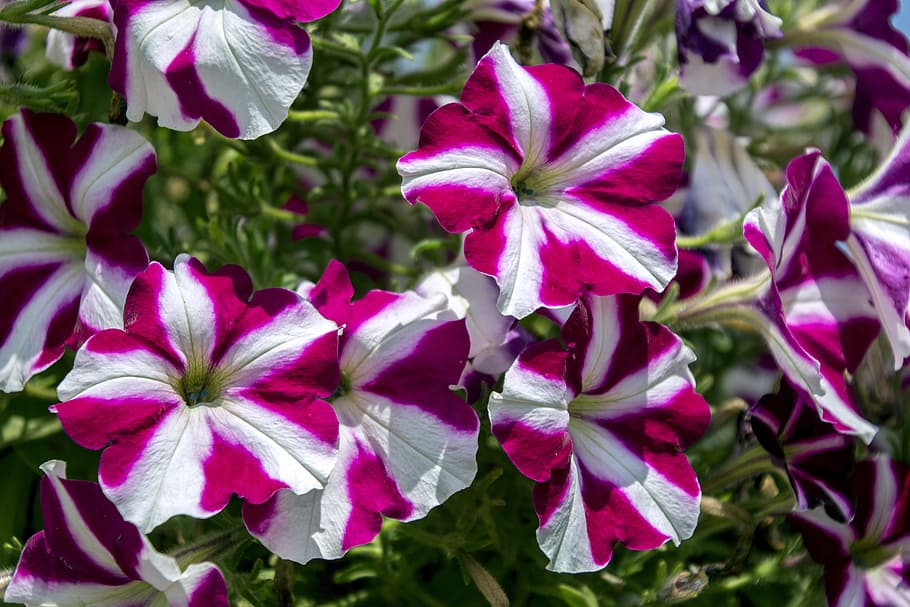
Reading Question #3
Which term describes the expression of both alleles for the same characteristic in a heterozygote?
A. Dominance
B. Recessiveness
C. Incomplete dominance
D. Codominance
Research Connection: Insecticide Resistance
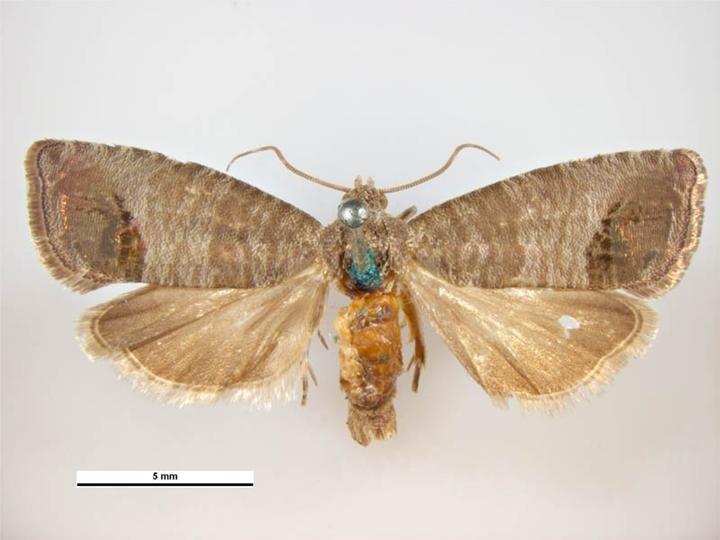
There is a longstanding battle between insecticide effectiveness and resistance evolution. For over a century, people have noticed how easily insects can develop resistance when an insecticide fails to kill the entire population, thereby selecting resistant individuals. Studies have found different patterns of resistance inheritance across various species (Sudo et al., 2017). For example, deltamethrin insecticide resistance in codling moths (Cydia pomonella) is expressed as an incompletely recessive trait (Bouvier et al., 2001).
Studies have also found that the dominance of insecticide resistance varies based on several factors of the insect’s environment. For example, the common house mosquito (Culex pipiens) showed dominance levels varying from almost complete dominance to almost recessive based on the type of insecticide and characteristics of their environment (food, water, and properties of the cup they were housed in) (Bourguet, Prout, & Raymond, 1996).
Multiple Alleles
Mendel implied that only two alleles, one dominant and one recessive, could exist for a given gene. We now know that this is an oversimplification. Although individual humans (and all diploid organisms) can only have two alleles for a given gene, multiple alleles may exist at the population level such that many combinations of two alleles are observed. Note that when many alleles exist for the same gene, the convention is to denote the most common phenotype or genotype among wild animals as the wild type (often abbreviated “+”); this is considered the standard or norm. All other phenotypes or genotypes are considered variants of this standard, meaning that they deviate from the wild type. The variant may be recessive or dominant to the wild-type allele.
An example of multiple alleles is coat color in rabbits (Figure 13.4). Here, four alleles exist for the c gene. The wild-type version, C+C+, is expressed as brown fur. The chinchilla phenotype, cchcch, is expressed as black-tipped white fur. The Himalayan phenotype, chch, has black fur on the extremities and white fur elsewhere. Finally, the albino, or “colorless” phenotype, cc, is expressed as white fur. In cases of multiple alleles, dominance hierarchies can exist. In this case, the wild-type allele is dominant over all the others, chinchilla is incompletely dominant over Himalayan and albino, and Himalayan is dominant over albino. This hierarchy, or allelic series, was revealed by observing the phenotypes of each possible heterozygote offspring.
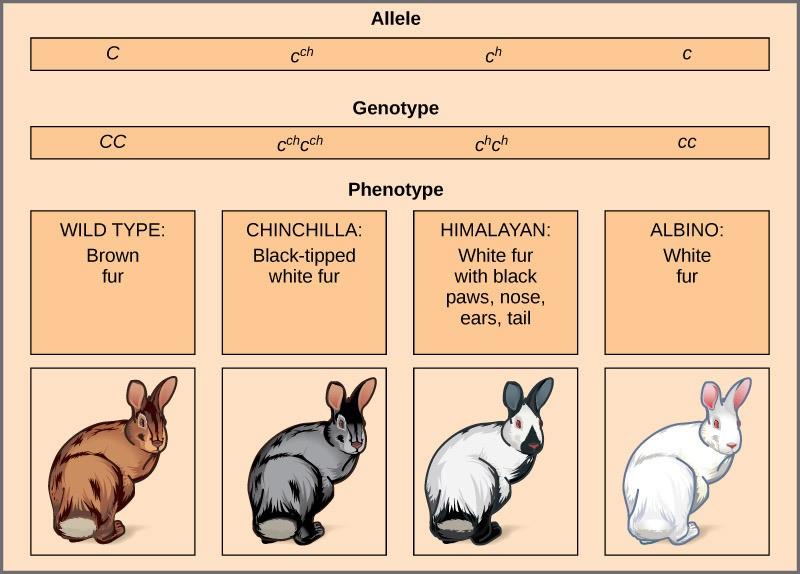
The complete dominance of a wild-type phenotype over all other mutants often occurs as an effect of the “dosage” of a specific gene product, such that the wild-type allele supplies the correct amount of gene product, whereas the mutant alleles cannot. For the allelic series in rabbits, the wild-type allele may supply a given dosage of fur pigment, whereas the mutants supply a lesser dosage or none at all. Interestingly, the Himalayan phenotype is the result of an allele that produces a temperature-sensitive gene product that only produces pigment in the cooler extremities of the rabbit’s body.
Alternatively, one mutant allele can be dominant over all other phenotypes, including the wild type. This may occur when the mutant allele somehow interferes with the genetic message, so even a heterozygote with one wild-type allele copy expresses the mutant phenotype. One way in which the mutant allele can interfere is by enhancing the function of the wild-type gene product or changing its distribution in the body. One example of this is the Antennapedia mutation in Drosophila (Figure 13.5). In this case, the mutant allele expands the gene product’s distribution; as a result, the Antennapedia heterozygote develops legs on its head where its antennae should be.
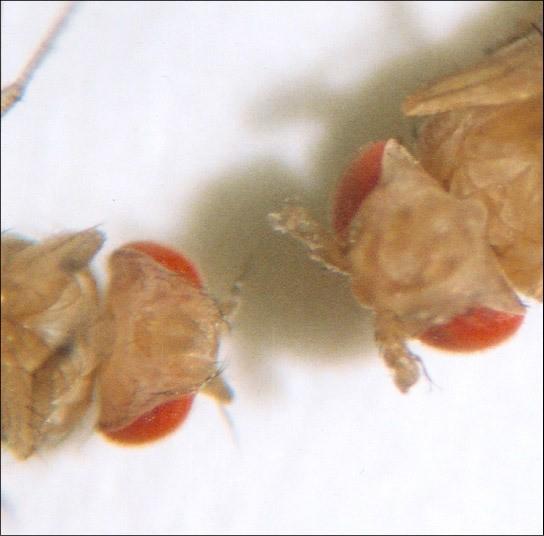
Reading Question #4
Which of the following statements about the rabbit coat color alleles is true?
A. The wild-type allele is dominant over chinchilla and Himalayan alleles.
B. The albino allele is dominant over all other alleles.
C. The chinchilla allele is dominant over the wild-type and Himalayan alleles.
D. The Himalayan allele is recessive to all other alleles.
Evolution Connection: Multiple Alleles Confer Drug Resistance in the Malaria Parasite
Malaria is a parasitic disease in humans that is transmitted by infected female mosquitoes, including Anopheles gambiae (Figure 13.6a), and is characterized by cyclic high fevers, chills, flu-like symptoms, and severe anemia. Plasmodium falciparum and P. vivax are the most common causative agents of malaria, and P. falciparum is the most deadly (Figure 13.6b). When promptly and correctly treated, P. falciparum malaria has a mortality rate of 0.1%. However, in some parts of the world, the parasite has evolved resistance to commonly used malaria treatments, so the most effective malarial treatments can vary by geographic region.
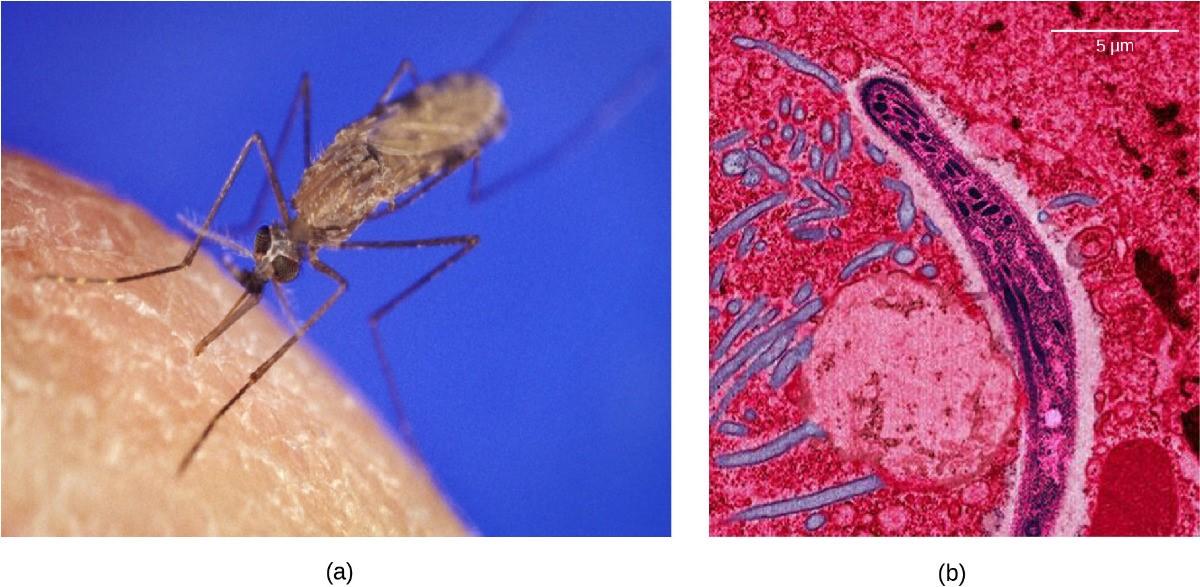
In Southeast Asia, Africa, and South America, P. falciparum has developed resistance to the anti-malarial drugs chloroquine, mefloquine, and sulfadoxine-pyrimethamine. P. falciparum, a haploid during the life stage in which it is infectious to humans, has evolved multiple drug-resistant mutant alleles of the dhps gene. Varying degrees of sulfadoxine resistance are associated with each of these alleles. Being haploid, P. falciparum needs only one drug-resistant allele to express this trait.
In Southeast Asia, different sulfadoxine-resistant alleles of the dhps gene are localized to different geographic regions. This is a common evolutionary phenomenon that occurs because drug-resistant mutants arise in a population and interbreed with other P. falciparum isolates in close proximity. Sulfadoxine-resistant parasites cause considerable human hardship in regions where this drug is widely used as an over-the-counter malaria remedy. As is common with pathogens that multiply to large numbers within an infection cycle, P. falciparum evolves relatively rapidly (over a decade or so) in response to the selective pressure of commonly used antimalarial drugs. For this reason, scientists must constantly work to develop new drugs or drug combinations to combat the worldwide malaria burden (Vinayak et al., 2010).
Lethality
A large proportion of genes in an individual’s genome are essential for survival. Occasionally, a nonfunctional allele for an essential gene can arise by mutation and be transmitted in a population as long as individuals with this allele also have a wild-type, functional copy. The wild-type allele functions at a capacity sufficient to sustain life and is therefore considered to be dominant over the nonfunctional allele. However, consider two heterozygous parents that have a genotype of wild type/nonfunctional mutant for a hypothetical essential gene. In one-quarter of their offspring, we would expect to observe homozygous recessive individuals for the nonfunctional allele. Because the gene is essential, these individuals might fail to develop past fertilization, die in utero, or die later in life, depending on what life stage requires this gene. An inheritance pattern in which an allele is only lethal in the homozygous form and in which the heterozygote may be normal or have some altered nonlethal phenotype is called recessive lethal.
For crosses between heterozygous individuals with a recessive lethal allele that causes death before birth when homozygous, only wild-type homozygotes and heterozygotes would be observed. The genotypic ratio would therefore be 2:1. In other instances, the recessive lethal allele might also exhibit a dominant (but not lethal) phenotype in the heterozygote. For instance, the recessive lethal Curly allele in Drosophila affects wing shape in the heterozygote form but is lethal in the homozygote.
A single copy of the wild-type allele is not always sufficient for normal functioning or even survival. The dominant lethal inheritance pattern is one in which an allele is lethal both in the homozygote and the heterozygote; this allele can only be transmitted if the lethality phenotype occurs after reproductive age. Individuals with mutations that result in dominant lethal alleles fail to survive even in the heterozygote form. Dominant lethal alleles are very rare because, as you might expect, the allele only lasts one generation and is not transmitted. However, just as the recessive lethal allele might not immediately manifest the phenotype of death, dominant lethal alleles also might not be expressed until adulthood. Once the individual reaches reproductive age, the allele may be unknowingly passed on, resulting in a delayed death in both generations. An example of this in humans is Huntington disease, in which the nervous system gradually degrades. People who are heterozygous for the dominant Huntington allele (Hh) will inevitably develop the fatal disease. However, the onset of Huntington’s disease may not occur until age 40; at this point, the afflicted persons may have already passed the allele to 50% of their offspring.
Epistasis
Mendel’s studies in pea plants implied that the sum of an individual’s phenotype was controlled by genes such that every characteristic was distinctly and completely controlled by a single gene. In fact, single observable characteristics are almost always under the influence of multiple genes (each with two or more alleles) acting in unison. For example, at least eight genes contribute to eye color in humans.
Link to Learning
Eye color in humans is determined by multiple genes. Use the Eye Color Calculator to predict the eye color of children from parental eye color.
In some cases, several genes can contribute to aspects of a common phenotype without their gene products ever directly interacting. In the case of organ development, for instance, genes may be expressed sequentially, with each gene adding to the complexity and specificity of the organ. Genes may function in complementary or synergistic fashions, such that two or more genes need to be expressed simultaneously to affect a phenotype. Genes may also oppose each other, with one gene modifying the expression of another.
In epistasis, the interaction between genes is antagonistic, such that one gene mask or interferes with the expression of another. “Epistasis” is a word composed of Greek roots that mean “standing upon.” The alleles that are being masked or silenced are said to be hypostatic to the epistatic alleles that are doing the masking. Often the biochemical basis of epistasis is a gene pathway in which the expression of one gene is dependent on the function of a gene that precedes or follows it in the pathway.
An example of epistasis is pigmentation in mice. The wild-type coat color, agouti (AA), is dominant to solid-colored fur (aa). However, a separate gene (C) is necessary for pigment production. A mouse with a recessive c allele at this locus is unable to produce pigment and is albino regardless of the allele present at locus A (Figure 13.7). Therefore, the genotypes AAcc, Aacc, and aacc all produce the same albino phenotype. A cross between heterozygotes for both genes (AaCc x AaCc) would generate offspring with a phenotypic ratio of 9 agouti:3 solid color:4 albino (Figure 13.7). In this case, the C gene is epistatic to the A gene.
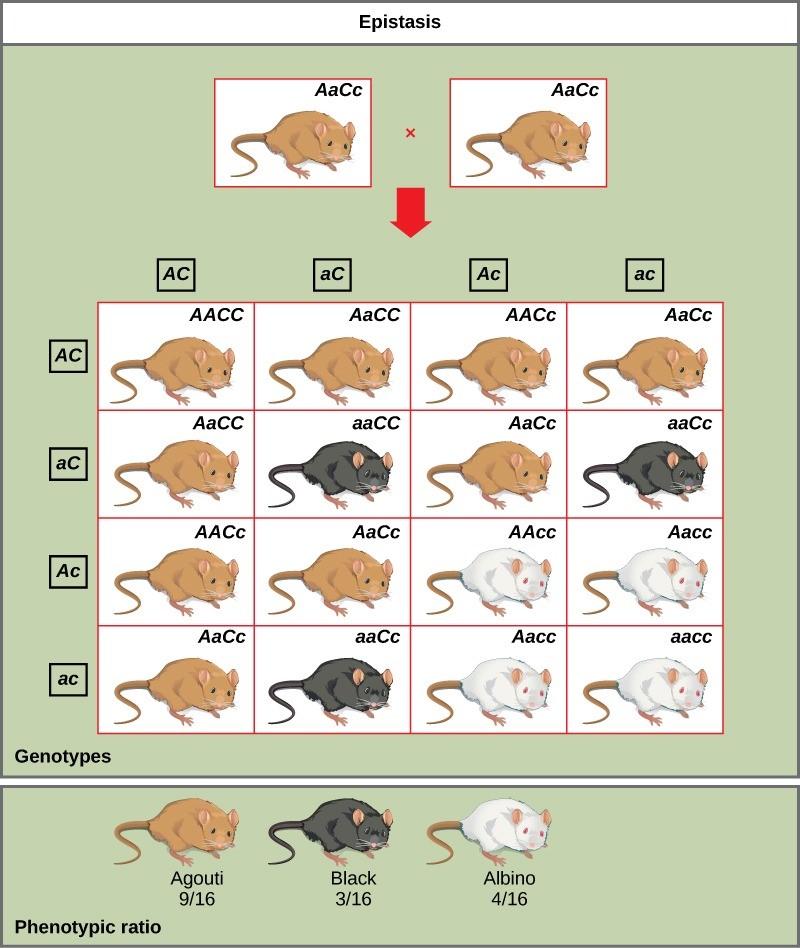
Epistasis can also occur when a dominant allele masks expression at a separate gene. Fruit color in summer squash is expressed in this way. The homozygous recessive expression of the W gene (ww) coupled with the homozygous dominant or heterozygous expression of the Y gene (YY or Yy) generates yellow fruit, and the wwyy genotype produces green fruit. However, if a dominant copy of the W gene is present in the homozygous or heterozygous form, the summer squash will produce white fruit regardless of the Y alleles. A cross between white heterozygotes for both genes (WwYy × WwYy) would produce offspring with a phenotypic ratio of 12 white:3 yellow:1 green.
Epistasis can be reciprocal such that either gene expresses the same phenotype when present in the dominant (or recessive) form. In the shepherd’s purse plant (Capsella bursa-pastoris), the characteristic of seed shape is controlled by two genes in a dominant epistatic relationship. When genes A and B are both homozygous recessive (aabb), the seeds are ovoid. If the dominant allele for either of these genes is present, the result is triangular seeds. Every possible genotype other than aabb results in triangular seeds, and a cross between heterozygotes for both genes (AaBb x AaBb) would yield offspring with a phenotypic ratio of 15 triangular:1 ovoid.
As you work through genetics problems, remember that any characteristic that results in a phenotypic ratio that totals 16 is typical of a two-gene interaction. Recall the phenotypic inheritance pattern for Mendel’s dihybrid cross, which considered two noninteracting genes 9:3:3:1. Similarly; we expect interacting gene pairs to exhibit ratios expressed as 16 parts. We assume the interacting genes are not linked; they are still assorting independently into gametes.
Reading Question #5
Which of the following statements best describes the concept of epistasis?
A. Multiple genes working together to control a single phenotype.
B. The interaction between genes in which one gene masks or interferes with the expression of another.
C. Genes expressed sequentially in a pathway, adding complexity and specificity to an organ.
D. Genes functioning in complementary or synergistic fashions, requiring simultaneous expression to affect a phenotype.
References and Acknowledgements
Bourguet, D., Prout, M., and Raymond, M. (1996). Dominance of insecticide resistance presents a plastic response. Genetics 143(1):407-16. doi: 10.1093/genetics/143.1.407
Bouvier, JC., Buès, R., Boivin, T. , Boudinhon, L., Beslay, D., and Sauphanor, B. (2001). Deltamethrin resistance in the codling moth (Lepidoptera: Tortricidae): inheritance and number of genes involved. Heredity 87, 456–462. https://doi.org/10.1046/j.1365-2540.2001.00928.x
Sudo, M., Takahashi, D., Andow, D.A., Suzuki, Y., and Yamanaka, T. (2017). Optimal management strategy of insecticide resistance under various insect life histories: Heterogeneous timing of selection and interpatch dispersal. Evolutionary Applications 11(2):271-283. doi: 10.1111/eva.12550
Vinayak, S., Tauqeer Alam, M., Mixson-Hayden, T., McCollum, A.M., Semet, R. … Udhayakumar, V. (2010). Origin and Evolution of Sulfadoxine Resistant Plasmodium falciparum Public Library of Science Pathogens 6(3):
Text Adapated from Lumen Learning, Biology for majors I. https://courses.lumenlearning.com/wm-biology1/chapter/non-mendelian-inheritance/