3 Chapter 3: M Phase
Lisa Limeri; Shifath Bin Syed; and rocksher
Learning Objectives
By the end of this section, you will be able to do the following:
- Explain why chromosome replication has to occur before mitosis, in interphase.
- Explain the differences between somatic cells and germ cells. Describe the outcomes of cell division between these two categories of cells.
- Given a micrograph or drawing of a cell you’ve never seen before that is undergoing mitosis, explain what is currently happening to the chromosomes.
Introduction
In the last chapter, we learned in detail about the cell cycle: an orderly sequence of events that describes the stages of a cell’s life, from the division of a single parent cell to the production of two new genetically identical daughter cells. We learned that a critical part of the cell cycle is the M phase, in which mitosis and cytokinesis occur. Mitosis is the process of duplicated chromosomes being aligned, separated, and moving into two new, identical daughter nuclei. Today we will dive into the details of mitosis and cytokinesis. Before we can learn about how chromosomes move and separate, we must first learn about the structure and organization of chromosomes.
Prokaryotic and Eukaryotic Genomes
A cell’s DNA, packaged as a double-stranded DNA molecule, is called its genome. In prokaryotes, the genome comprises a single, double-stranded DNA molecule in the form of a loop or circle (Figure 3.1). The DNA is clustered in a region in the cell called the nucleoid (but this is not bound by a nuclear membrane, like the nucleus of eukaryotic cells).
In eukaryotes, the genome consists of several double-stranded linear DNA molecules (Fig 3.1). (Figure 3.3). Each linear strand of DNA is so long that it must be coiled to be stored efficiently. This condensed, coiled structure of DNA is called a chromosome (more on this below). Chromosomes are stored in the nucleus, which is the primary feature distinguishing eukaryotic and prokaryotic cells. Most eukaryotic organisms have more than one copy of each gene. This is adaptive because it helps protect the organism against DNA damage. Think back to when we discussed genes that regulate the cell cycle in the last module: if one copy of the gene that codes for the p53 protein is damaged, but the other is intact, the cell may still be able to generate the p53 protein. The number of copies of each type of chromosome is called the ploidy. Humans have two copies of each type of chromosome, making us diploids (di = 2). Chromosomes that code for the same sets of genes are called homologous chromosomes. Humans have 23 chromosomes that code for unique sets of genes, and two copies of each of these chromosomes, making the total number of chromosomes 46 (another way to say that is that humans have 23 pairs of homologous chromosomes).
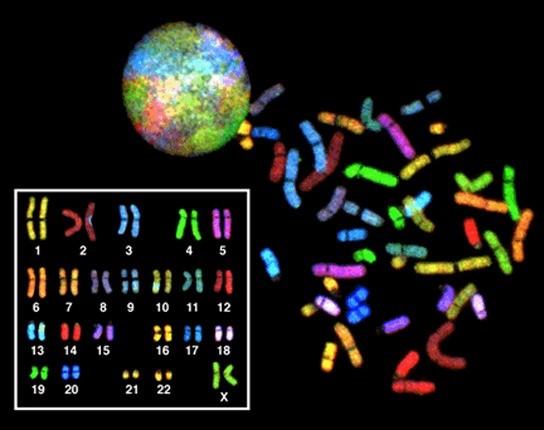
Reading Check #1
Which of the following best describes the genome organization in prokaryotes?
A. Multiple linear DNA molecules stored in the nucleus.
B. Single circular DNA molecule stored in the nucleoid.
C. Multiple circular DNA molecules stored in the nucleus.
D. Single linear DNA molecule stored in the nucleoid.
Chromosomes are Structures of Compact DNA in eukaryotes
If the DNA from all 46 chromosomes in a human cell nucleus were laid out end-to-end, it would measure approximately two meters; however, its diameter would be only 2 nm! Since a typical human cell is about 10 µm (100,000 cells lined up to equal one meter), DNA must be tightly packaged to fit in the cell’s nucleus. At the same time, it must also be readily accessible for the genes to be expressed. For this reason, the long strands of DNA are condensed into compact chromosomes during certain stages of the cell cycle.
In the first level of compaction, short stretches of the DNA double helix wrap around a core of eight histone proteins at regular intervals along the entire length of the chromosome. The DNA histone complex is called chromatin. The beadlike, histone DNA complex is called a nucleosome, and DNA connecting the nucleosomes is called linker DNA. A DNA molecule in this form is about seven times shorter than the double helix without the histones, and the beads are about 10 nm in diameter, in contrast with the 2nm diameter of a DNA double helix.
DNA replicates in the S phase of interphase, which is not a part of mitosis, but must always precede it. After replication, the chromosomes are composed of two linked sister chromatids. When fully compact, the pairs of identically packed chromosomes are bound to each other by cohesin proteins. The connection between the sister chromatids is closest in a region called the centromere. The conjoined sister chromatids, with a diameter of about 1 µm, are visible under a light microscope. The centromeric region is highly condensed and thus will appear as a constricted area.
Reading Check #2
What is the principal role of histone proteins in the packing of chromatin?
A. To carry out DNA replication while the cell cycle is in the S phase.
B. To pack DNA into a double-stranded structure.
C. To organize DNA into a higher-order structure and produce nucleosomes.
D. To transport chromosomes during mitosis.
Mitotic Phase
The mitotic phase is a multistep process during which the duplicated chromosomes are aligned, separated, and moved into two new, identical daughter cells. The first portion of the mitotic phase is called mitosis, or nuclear division. The second portion of the mitotic phase (and often viewed as a process separate from and following mitosis) is called cytokinesis—the physical separation of the cytoplasmic components into the two daughter cells.
Mitosis
We describe the events of mitosis as a series of phases—prophase, prometaphase, metaphase, anaphase, and telophase—that result in the division of the cell nucleus (Fig 3.2).
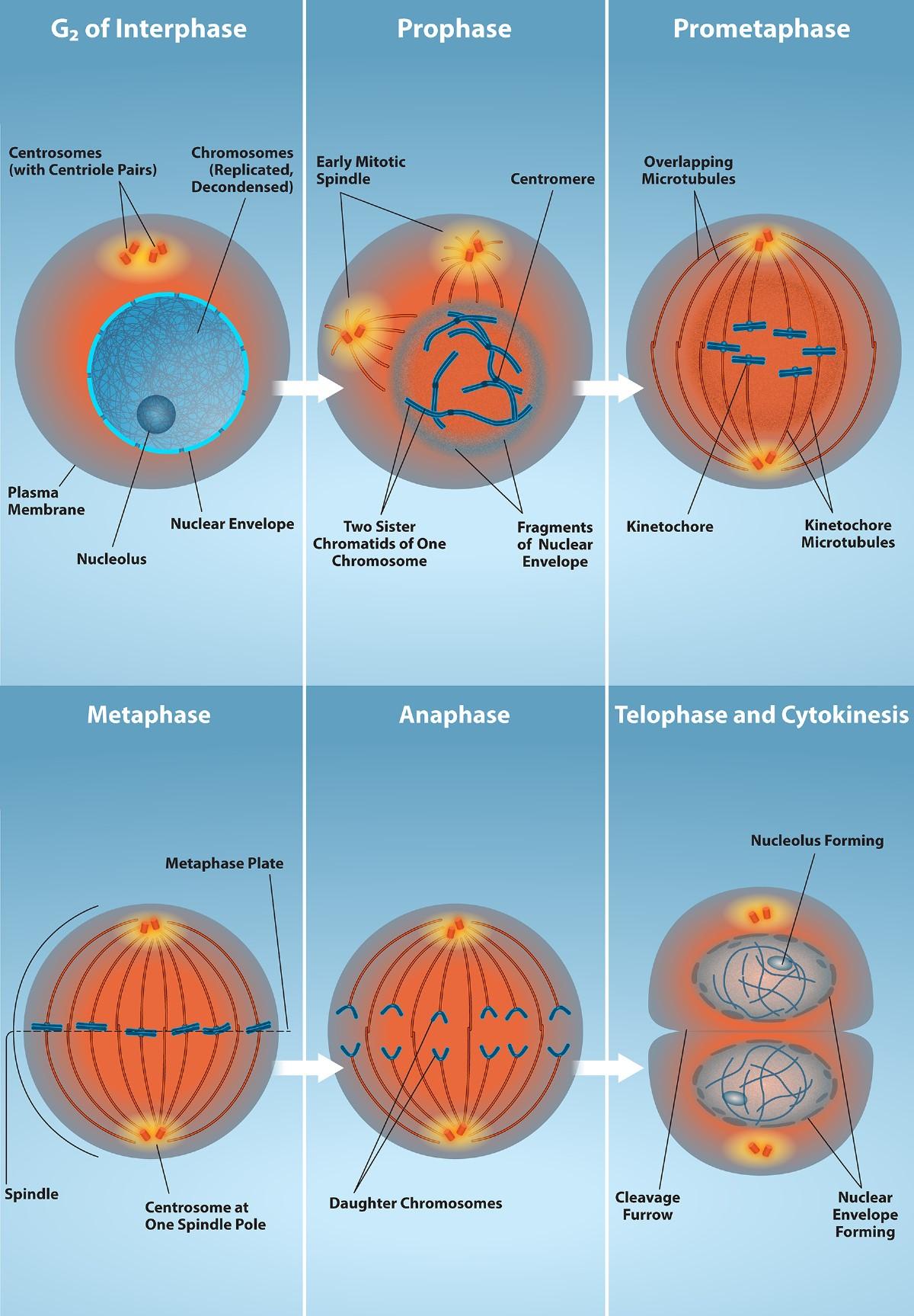
Prophase (the “first phase”): the nuclear envelope starts to dissolve, and the membranous organelles (such as the Golgi apparatus and the endoplasmic reticulum) fragment and disperse toward the periphery of the cell. The nucleolus also disappears (disperses), and the centrosomes begin to move to opposite poles of the cell. A pair of centrioles joined together form a centrosome. Microtubules forming the mitotic spindle extend between the centrosomes, pushing them farther apart as the microtubule fibers lengthen. The sister chromatids begin to coil more tightly with the aid of condensin proteins. They are becoming dense enough to be visible under a light microscope.
Prometaphase (the “first change phase”): Many processes that began in prophase continue to advance. The remnants of the nuclear envelope fragment further, and the mitotic spindle continues to develop as more microtubules assemble and stretch across the length of the former nuclear area. Chromosomes become even more condensed and discrete. Each sister chromatid develops a kinetochore protein structure in its centromeric region (Fig 3.3). The proteins of the kinetochore attract and bind to the mitotic spindle microtubules. As the spindle microtubules extend from the centrosomes, some of these microtubules come into contact with and firmly bind to the kinetochores. Once a mitotic fiber attaches to a chromosome, the chromosome will be oriented until the kinetochores of sister chromatids face the opposite poles. Eventually, all the sister chromatids will be attached via their kinetochores to microtubules from opposing poles. Spindle microtubules that do not engage the chromosomes are called polar microtubules. These microtubules overlap each other midway between the two poles and contribute to cell elongation. Astral microtubules are located near the poles, aid in spindle orientation, and are required for the regulation of mitosis.
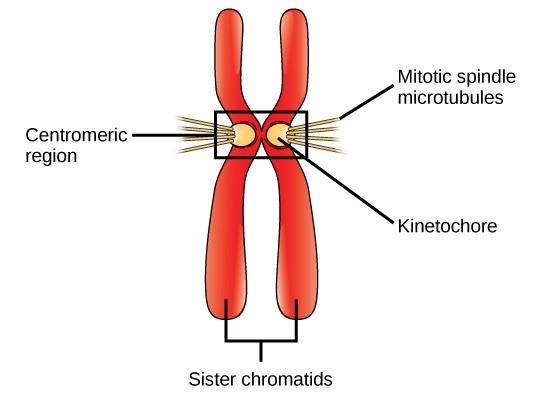
Metaphase (the “change phase”): All the chromosomes are aligned in a plane called the metaphase plate, or the equatorial plane, roughly midway between the two poles of the cell. The sister chromatids are still tightly attached to each other by cohesin proteins. At this time, the chromosomes are maximally condensed. At this time, the M phase check point occurs to ensure that all the chromosomes are intact and correctly aligned.
Anaphase (“upward phase”): The cohesin proteins degrade, and the sister chromatids separate at the centromere. Each chromatid, now called a single chromosome once separated, is pulled rapidly toward the centrosome to which its microtubule is attached. The cell becomes visibly elongated (oval-shaped) as the polar microtubules slide against each other at the metaphase plate, where they overlap.
Telophase (the “distance phase”): the chromosomes reach the opposite poles and begin to decondense (unravel), relaxing once again into a stretched-out chromatin configuration. The mitotic spindles are depolymerized into tubulin monomers that will be used to assemble cytoskeletal components for each daughter cell. Nuclear envelopes form around the chromosomes, and nucleosomes appear within the nuclear area.
Reading Check #3
During which phase of mitosis do the sister chromatids separate and move towards opposite poles of the cell?
A. Prophase
B. Prometaphase
C. Metaphase
D. Anaphase
Reading Check #4
In animal cells, which structure forms during cytokinesis to initiate the physical separation of the cytoplasm?
A. Cleavage furrow
B. Cell plate
C. Contractile ring
D. Phragmoplast
Cytokinesis
Cytokinesis, or “cell motion,” is the second main stage of the mitotic phase, during which cell division is completed via the physical separation of the cytoplasmic components into two daughter cells. Depending on the organism, cytokinesis may or may not take place following mitosis. Although the stages of mitosis are similar for most eukaryotes, the process of cytokinesis is quite different for eukaryotes that have cell walls, such as plant cells.
In animal cells, cytokinesis typically starts during late anaphase. A contractile ring composed of actin filaments forms just inside the plasma membrane at the former metaphase plate. The actin filaments pull the equator of the cell inward, forming a fissure. This fissure is called the cleavage furrow. The furrow deepens as the actin ring contracts, and eventually, the membrane is cleaved in two (Fig 3.4).
In plant cells, a new cell wall must form between the daughter cells. During interphase, the Golgi apparatus accumulates enzymes, structural proteins, and glucose molecules before breaking into vesicles and dispersing throughout the dividing cell. During telophase, these Golgi vesicles are transported on microtubules to form a phragmoplast (a vesicular structure) at the metaphase plate. The vesicles fuse and coalesce from the center toward the cell walls; this structure is called a cell plate. As more vesicles fuse, the cell plate enlarges until it merges with the cell walls at the cell periphery. Enzymes use the glucose that has accumulated between the membrane layers to build a new cell wall. The Golgi membranes become parts of the plasma membrane on either side of the new cell wall (Fig 3.4).

Evolution Connection: Mitotic Spindle Apparatus
The precise timing and formation of the mitotic spindle are critical to the success of eukaryotic cell division. Prokaryotic cells, on the other hand, do not undergo mitosis (they do not have linear chromosomes) and therefore do not need a mitotic spindle. However, the FtsZ protein that plays such a vital role in prokaryotic cytokinesis is structurally and functionally very similar to tubulin, the building block of the microtubules that make up the mitotic spindle fibers that are necessary for eukaryotic nuclear division. FtsZ proteins can form filaments, rings, and other three-dimensional structures resembling tubulin forms microtubules, centrioles, and various cytoskeletal components. In addition, both FtsZ and tubulin employ the same energy source, GTP (guanosine triphosphate), to rapidly assemble and disassemble complex structures.
FtsZ and tubulin are considered homologous structures derived from common evolutionary origins. In this example, FtsZ is the ancestor protein of tubulin (an evolutionarily derived protein). While both proteins are found in extant organisms, tubulin function has evolved and diversified tremendously since evolving from its FtsZ prokaryotic origin. A survey of mitotic assembly components found in present-day unicellular eukaryotes reveals crucial intermediary steps to the complex membrane-enclosed genomes of multicellular eukaryotes.
Research Connection: Evolution from Prokaryotes to Eukaryotes
Eukaryotes are generally thought to have appeared approximately 1-2 billion years after prokaryotes (ScienceDaily, 2022; Warring, 2016). Recently, a research team from Japan identified an organism that appears to be evolutionary intermediate between prokaryotes and eukaryotes. This group of organisms is known as Asgard archaea, referencing Norse mythology. The research team identified a protein in Asgard archaea, similar to eukaryotic tubulin, which they named OdinTubulin (ScienceDaily, 2022). Read more about the Asgard archaea study on ScienceDaily.
Reading Check #5
What would be the result of cells undergoing mitosis but did not replicate their chromosomes first?
A. Daughter cells with the original number of chromosomes.
B. Daughter cells with half the original number of chromosomes.
C. Sister chromatids will stay together during separation of homologous pairs.
D. Some daughter cells will have the correct number of chromosomes and others will have half.
References and Acknowledgments
Adapted from Clark, M.A., Douglas, M., and Choi, J. (2018). Biology 2e. OpenStax. Retrieved from https://openstax.org/books/biology-2e/pages/1-introduction
ScienceDaily. (2022, April 20). Scientists discover potential key missing link protein bridging eukaryotes and prokaryotes. Retrieved August 17, 2022, from https://www.sciencedaily.com/releases/2022/04/220420092142.html
Warring, S. (2016, February 24). Cells Living in cells Arizona State University. Retrieved August 17, 2022, from https://askabiologist.asu.edu/explore/cells-living-in-cells