29 Chapter 29: Asexual and Sexual Reproduction
Anastasia Chouvalova
Learning Objectives
By the end of this chapter, you should be able to
29.1 Describe the four modes of asexual reproduction and where you might expect to find each mode: fragmentation, budding, fission, and parthenogenesis.
29.2 Describe advantages and disadvantages of external fertilization, internal fertilization, sexual reproduction, asexual reproduction, and self-fertilization.
Animals produce offspring through asexual and/or sexual reproduction. Both methods have advantages and disadvantages. Asexual reproduction produces offspring that are genetically identical to the parent because the offspring are all clones of the original parent. A single individual can produce offspring asexually and large numbers of offspring can be produced quickly. In a stable or predictable environment, asexual reproduction is an effective means of reproduction because all the offspring will be adapted to that environment. In an unstable or unpredictable environment asexually-reproducing species may be at a disadvantage because all the offspring are genetically identical and may not have the genetic variation to survive in new or different conditions. On the other hand, the rapid rates of asexual reproduction may allow for a speedy response to environmental changes if individuals have mutations. An additional advantage of asexual reproduction is that colonization of new habitats may be easier when an individual does not need to find a mate to reproduce.
During sexual reproduction the genetic material of two individuals is combined to produce genetically diverse offspring that differ from their parents. The genetic diversity of sexually produced offspring is thought to give species a better chance of surviving in an unpredictable or changing environment. Species that reproduce sexually must maintain two different types of individuals, males and females, which can limit the ability to colonize new habitats as both sexes must be present.
Reading Question #1
Which of the following is not an advantage of asexual reproduction?
A. Easier colonization of new habitats
B. Fast rate of offspring production
C. Many offspring produced
D. A more diverse gene pool
Asexual Reproduction
Asexual reproduction occurs in prokaryotic microorganisms (bacteria) and in some eukaryotic single-celled and multi-celled organisms. There are a number of ways that animals reproduce asexually – fission, budding, fragmentation, and parthenogenesis.
Fission
Fission, also called binary fission, occurs in prokaryotic microorganisms and in some invertebrate, multi-celled organisms. After a period of growth, an organism splits into two separate organisms. Some unicellular eukaryotic organisms undergo binary fission by mitosis. In other organisms, part of the individual separates and forms a second individual. This process occurs, for example, in many asteroid echinoderms through splitting of the central disk. Some sea anemones and some coral polyps (Figure 25.1) also reproduce through fission.
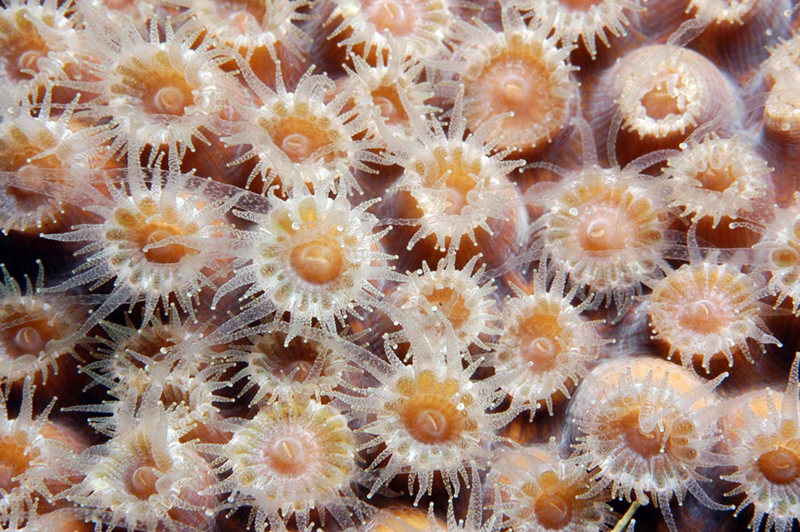
Budding
Budding is a form of asexual reproduction that results from the outgrowth of a part of a cell or body region leading to a separation from the original organism into two individuals. Budding occurs commonly in some invertebrate animals such as corals and hydras. In hydras, a bud forms that develops into an adult and breaks away from the main body, as illustrated in Figure 25.2, whereas in coral budding, the bud does not detach and multiplies as part of a new colony. Visit this link to view a video of hydra budding.
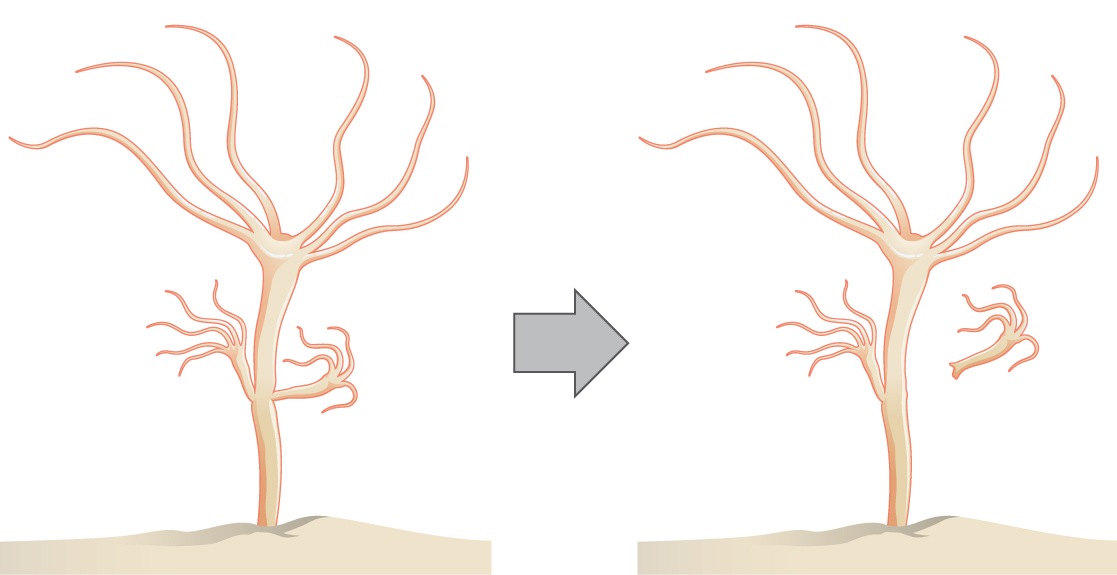
Fragmentation
Fragmentation is the breaking of the body into two parts with subsequent regeneration. If the animal is capable of fragmentation, and the part is big enough, a separate individual will regrow.
For example, in many sea stars, asexual reproduction is accomplished by fragmentation. Figure 25.3 illustrates a sea star for which an arm of the individual is broken off and regenerates a new sea star. Fisheries workers have been known to try to kill the sea stars eating their clam or oyster beds by cutting them in half and throwing them back into the ocean. Unfortunately for the workers, the two parts can each regenerate a new half, resulting in twice as many sea stars to prey upon the oysters and clams. Fragmentation also occurs in annelid worms, turbellarians, and poriferans.
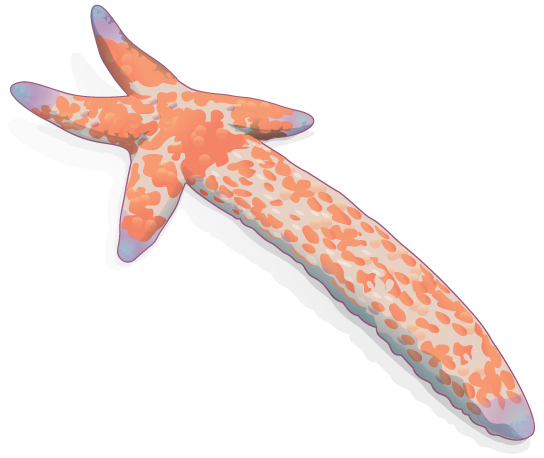
Note that in fragmentation, there is generally a noticeable difference in the size of the individuals, whereas in fission, two individuals of approximate size are formed.
Parthenogenesis
Parthenogenesis is a form of asexual reproduction where an egg develops into a complete individual without being fertilized. The resulting offspring can be either haploid or diploid, depending on the process and the species. Parthenogenesis occurs in invertebrates such as water fleas, rotifers, aphids, stick insects, some ants, wasps, and bees. Bees use parthenogenesis to produce haploid males (drones). If eggs are fertilized, diploid females develop, and if the fertilized eggs are fed a special diet (so called royal jelly), a queen is produced.
Some vertebrate animals—such as certain reptiles, amphibians, and fish—also reproduce through parthenogenesis. Although more common in plants, parthenogenesis has been observed in animal species that were segregated by sex in terrestrial or marine zoos. Two female Komodo dragons, a hammerhead shark, and a blacktop shark have produced parthenogenic young when the females have been isolated from males.
Reading Question #2
Match the following definitions of different types of asexual reproduction to their terms.
A) Fission B) Budding C) Fragmentation D) Parthenogenesis
- An unfertilized egg becomes a fully-functioning organism
- One body separates into two separate bodies.
- A portion of the parental organism splits into smaller parts.
- Some outgrowth of the parental organism produces the offspring.
Fertilization methods in sexual reproduction
Sexual reproduction starts with the combination of a sperm and an egg in a process called fertilization. This can occur either inside (internal fertilization) or outside (external fertilization) the body of the female. Humans provide an example of the former whereas seahorse reproduction is an example of the latter.
External Fertilization
External fertilization usually occurs in aquatic environments where both eggs and sperm are released into the water. After the sperm reaches the egg, fertilization takes place. Most external fertilization happens during the process of spawning where one or several females release their eggs and the male(s) release sperm in the same area, at the same time. The release of the reproductive material may be triggered by water temperature or the length of daylight. Nearly all fish spawn, as do crustaceans (such as crabs and shrimp), mollusks (such as oysters), squid, and echinoderms (such as sea urchins and sea cucumbers). Figure 25.4 shows salmon spawning in a shallow stream. Frogs, like those shown in Figure 25.5, corals, mollusks, and sea cucumbers also spawn.
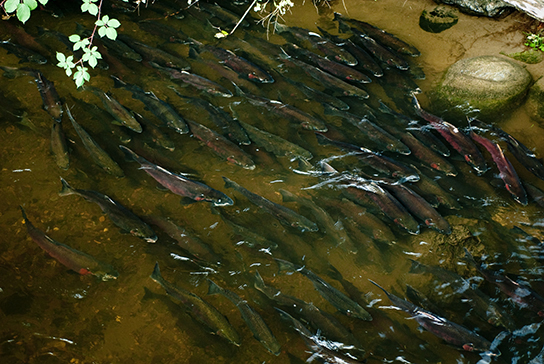
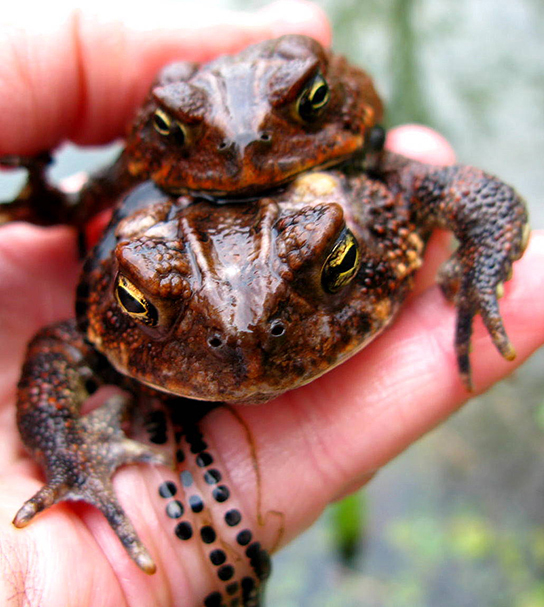
Pairs of fish that are not broadcast spawners may exhibit courtship behavior. This allows the female to select a particular male. The trigger for egg and sperm release (spawning) causes the egg and sperm to be placed in a small area, enhancing the possibility of fertilization.
External fertilization in an aquatic environment protects the eggs from drying out. Broadcast spawning can result in a greater mixture of the genes within a group, leading to higher genetic diversity and a greater chance of species survival in a hostile environment. For sessile aquatic organisms like sponges, broadcast spawning is the only mechanism for fertilization and colonization of new environments. The presence of the fertilized eggs and developing young in the water provides opportunities for predation resulting in a loss of offspring. Therefore, millions of eggs must be produced by individuals, and the offspring produced through this method must mature rapidly. The survival rate of eggs produced through broadcast spawning is low.
Reading Question #3
Which of the following species is least likely to use external fertilization?
A. Sea anemones
B. Turtles
C. Cod fish
D. American bullfrog
Internal Fertilization
Internal fertilization occurs most often in land-based animals, although some aquatic animals also use this method. There are three ways that offspring are produced following internal fertilization. In oviparity, fertilized eggs are laid outside the parent’s body and develop there, receiving nourishment from the yolk that is a part of the egg. This occurs in most bony fish, many reptiles, some cartilaginous fish, most amphibians, two mammals, and all birds. Reptiles and insects produce leathery eggs, while birds and turtles produce eggs with high concentrations of calcium carbonate in the shell, making them hard. Chicken eggs are an example of this second type.
In ovoviviparity, fertilized eggs are retained in the parent (usually the female), but the embryo obtains its nourishment from the egg’s yolk and the young are fully developed when they are hatched. This occurs in some bony fish (like the guppy Lebistes reticulatus), some sharks, some lizards, some snakes (such as the garter snake Thamnophis sirtalis), some vipers, and some invertebrate animals (like the Madagascar hissing cockroach Gromphadorhina portentosa).
In viviparity the young develop within the female, receiving nourishment from the parent’s blood through a placenta. The offspring develops in the female and is born alive. This occurs in most mammals, some cartilaginous fish, and a few reptiles.
Internal fertilization has the advantage of protecting the fertilized egg from dehydration on land. The embryo is isolated within the female, which limits predation on the young. Internal fertilization enhances the fertilization of eggs by a specific male. Fewer offspring are produced through this method, but their survival rate is higher than that for external fertilization.
Reading Question #4
The great white shark mother contains the fertilized egg and the yolk nourishes the embryo until it is mature enough to hatch. This is a description of:
A. Oviparity
B. Ovoviviparity
C. Viviparity
D. External fertilization
The Evolution of Reproduction
Once multicellular organisms evolved and developed specialized cells, some also developed tissues and organs with specialized functions. An early development in reproduction occurred in the Annelids. These organisms produce sperm and eggs from undifferentiated cells in their coelom and store them in that cavity. When the coelom becomes filled, the cells are released through an excretory opening or by the body splitting open. Reproductive organs evolved with the development of gonads that produce sperm and eggs. These cells went through meiosis, an adaptation of mitosis, which reduced the number of chromosomes in each reproductive cell by half, while increasing the number of cells through cell division.
Complete reproductive systems were developed in insects, with separate sexes. Sperm are made in testes and then travel through coiled tubes to the epididymis for storage. Eggs mature in the ovary. When they are released from the ovary, they travel to the uterine tubes for fertilization. Some insects have a specialized sac, called a spermatheca, which stores sperm for later use, sometimes up to a year. Fertilization can be timed with environmental or food conditions that are optimal for offspring survival.
Vertebrates have similar structures, with a few differences. Non-mammals, such as birds and reptiles, have a common body opening, called a cloaca, for the digestive, excretory and reproductive systems. Coupling between birds usually involves positioning the cloaca openings opposite each other for transfer of sperm. Mammals have separate openings for the systems in the female and a uterus for support of developing offspring. The uterus has two chambers in species that produce large numbers of offspring at a time, while species that produce one offspring, such as primates, have a single uterus.
Sperm transfer from the male to the female during reproduction ranges from releasing the sperm into the watery environment for external fertilization, to the joining of cloaca in birds, to the development of a penis for direct delivery into the female’s vagina in mammals.
Reading Question #5
Which of the following statements correctly describes external and internal fertilization in sexual reproduction? Select all that apply.
A. In internal fertilization, the offspring is fertilized outside of the parent’s body while in external fertilization, the offspring is fertilized inside of the parent’s body.
B. In internal fertilization, the main advantage is that the embryo is protected from extrinsic factors such as wind and lack of humidity.
C. In external fertilization, there tends to be less offspring produced.
D. Three examples of external fertilization are oviparity, ovoviviparity, and vivparity.
Sexual reproduction
Sexual reproduction was likely an early evolutionary innovation after the appearance of eukaryotic cells. It appears to have been very successful because most eukaryotes are able to reproduce sexually and, in many animals, it is the only mode of reproduction. And yet, scientists also recognize some real disadvantages to sexual reproduction. On the surface, creating offspring that are genetic clones of the parent appears to be a better system. If the parent organism is successfully occupying a habitat, offspring with the same traits should be similarly successful. There is also the obvious benefit to an organism that can produce offspring whenever circumstances are favorable by asexual budding, fragmentation, or by producing eggs asexually. These methods of reproduction do not require a partner with which to reproduce. Indeed, some organisms that lead a solitary lifestyle have retained the ability to reproduce asexually. In addition, in asexual populations, every individual is capable of reproduction. In sexual populations, the males are not producing the offspring themselves, so hypothetically an asexual population could grow twice as fast.
However, multicellular organisms that exclusively depend on asexual reproduction are exceedingly rare. Why are meiosis and sexual reproductive strategies so common? These are important (and as yet unanswered) questions in biology, even though they have been the focus of much research beginning in the latter half of the 20th century. There are several possible explanations, one of which is that the variation that sexual reproduction creates among offspring is very important to the survival and reproduction of the population. Thus, on average, a sexually reproducing population will leave more descendants than an otherwise similar asexually reproducing population. The only source of variation in asexual organisms is mutation. Mutations that take place during the formation of germ cell lines are also a source of variation in sexually reproducing organisms. However, in contrast to mutation during asexual reproduction, the mutations during sexual reproduction can be continually reshuffled from one generation to the next when different parents combine their unique genomes and the genes are mixed into different combinations by crossovers during prophase I and random assortment at metaphase I.
Sexual reproduction requires the union of two specialized cells, called gametes, each of which contains one set of chromosomes. When gametes unite, they form a zygote, or fertilized egg that contains two sets of chromosomes. (Note: Cells that contain one set of chromosomes are called haploid; cells containing two sets of chromosomes are called diploid.) If the reproductive cycle is to continue for any sexually reproducing species, then the diploid cell must somehow reduce its number of chromosome sets to produce haploid gametes; otherwise, the number of chromosome sets will double with every future round of fertilization. Therefore, sexual reproduction requires a nuclear division that reduces the number of chromosome sets by half.
Most animals and plants and many unicellular organisms are diploid and therefore have two sets of chromosomes. In each somatic cell of the organism (all cells of a multicellular organism except the gametes or reproductive cells), the nucleus contains two copies of each chromosome, called homologous chromosomes. Homologous chromosomes are matched pairs containing the same genes in identical locations along their lengths. Diploid organisms inherit one copy of each homologous chromosome from each genetic contributor.
Meiosis is the nuclear division that forms haploid cells from diploid cells, and it employs many of the same cellular mechanisms as mitosis. However, as you have learned, mitosis produces daughter cells whose nuclei are genetically identical to the original parent nucleus. In mitosis, both the parent and the daughter nuclei are at the same “ploidy level”—diploid in the case of most multicellular animals. Plants use mitosis to grow as sporophytes, and to grow and produce eggs and sperm as gametophytes; so they use mitosis for both haploid and diploid cells (as well as for all other ploidies). In meiosis, the starting nucleus is always diploid and the daughter nuclei that result are haploid. To achieve this reduction in chromosome number, meiosis consists of one round of chromosome replication followed by two rounds of nuclear division. Because many events that occur during each of the division stages are analogous to the events of mitosis, the same stage names are assigned. However, because there are two rounds of division, the major process and the stages are designated with a “I” or a “II.” Thus, meiosis I is the first round of meiotic division and consists of prophase I, prometaphase I, and so on. Likewise, Meiosis II (during which the second round of meiotic division takes place) includes prophase II, prometaphase II, and so on. We discussed meiosis (Figure 26.1) in great detail in BIOL 1403, so we will not spend as much time on it in BIOL 1404.
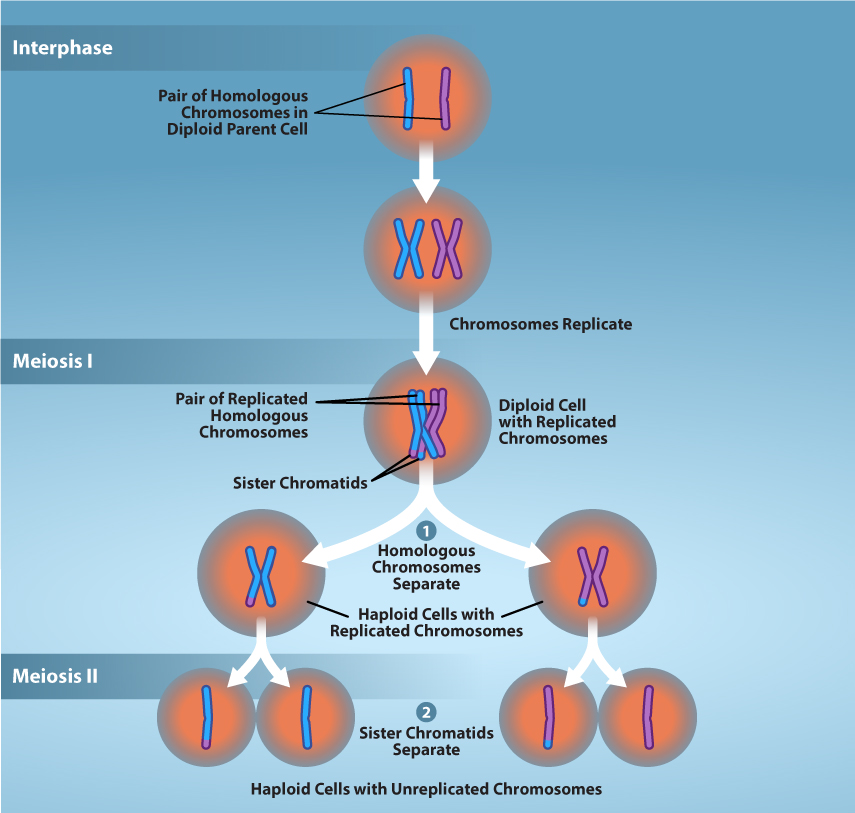
Evolution Connection
The Red Queen Hypothesis
Genetic variation is the outcome of sexual reproduction, but why are ongoing variations necessary, even under seemingly stable environmental conditions? Enter the Red Queen hypothesis, first proposed by Leigh Van Valen in 1973.3 The concept was named in reference to the Red Queen’s race in Lewis Carroll’s book, Through the Looking-Glass.
All species coevolve (evolve together) with other organisms. For example, predators evolve with their prey, and parasites evolve with their hosts. Each tiny advantage gained by favorable variation gives a species a reproductive edge over close competitors, predators, parasites, or even prey. However, survival of any given genotype or phenotype in a population is dependent on the reproductive fitness of other genotypes or phenotypes within a given species. The only method that will allow a coevolving species to maintain its own share of the resources is to also continually improve its fitness (the capacity of the members to produce more reproductively viable offspring relative to others within a species). As one species gains an advantage, this increases selection on the other species; they must also develop an advantage or they will be outcompeted. No single species progresses too far ahead because genetic variation among the progeny of sexual reproduction provides all species with a mechanism to improve rapidly. Species that cannot keep up become extinct. The Red Queen’s catchphrase was, “It takes all the running you can do to stay in the same place.” This is an apt description of coevolution between competing species.
Life Cycles of Sexually Reproducing Organisms
Fertilization and meiosis alternate in sexual life cycles. What happens between these two events depends on the organism’s “reproductive strategy.” The process of meiosis reduces the chromosome number by half. Fertilization, the joining of two haploid gametes, restores the diploid condition. Some organisms have a multicellular diploid stage that is most obvious and only produce haploid reproductive cells. Animals, including humans, have this type of life cycle. Other organisms, such as fungi, have a multicellular haploid stage that is most obvious. Plants and some algae have alternation of generations, in which they have multicellular diploid and haploid life stages that are apparent to different degrees depending on the group.
Nearly all animals employ a diploid-dominant life-cycle strategy in which the only haploid cells produced by the organism are the gametes. Early in the development of the embryo, specialized diploid cells, called germ cells, are produced within the gonads (such as the testes and ovaries). Germ cells are capable of mitosis to perpetuate the germ cell line and meiosis to produce haploid gametes. Once the haploid gametes are formed, they lose the ability to divide again. There is no multicellular haploid life stage. Fertilization occurs with the fusion of two gametes, usually from different individuals, restoring the diploid state (Figure 26.2).
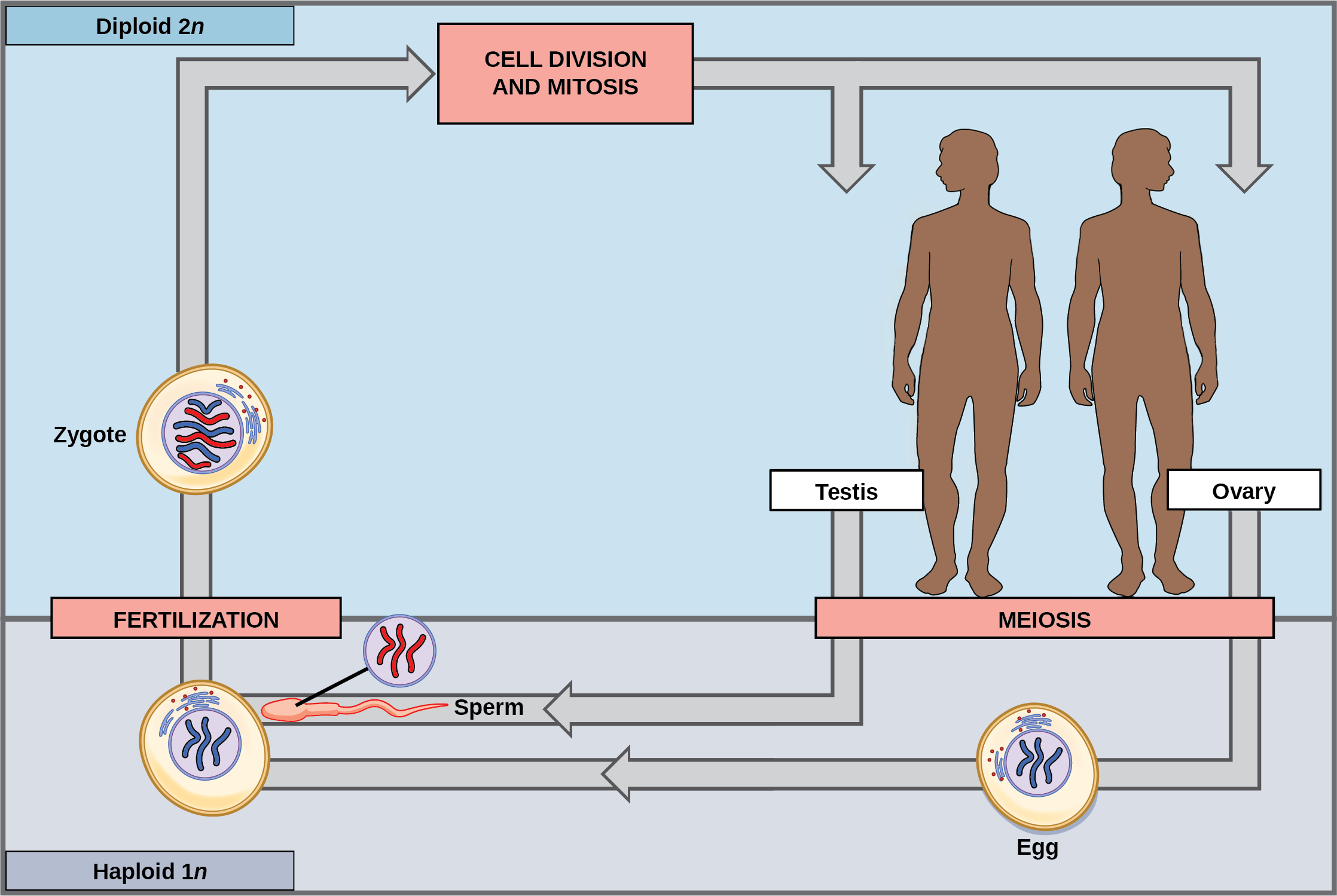
Most fungi and algae employ a life-cycle type in which the “body” of the organism—the ecologically important part of the life cycle—is haploid. The haploid cells that make up the tissues of the dominant multicellular stage are formed by mitosis. During sexual reproduction, specialized haploid cells from two individuals—designated the (+) and (−) mating types—join to form a diploid zygote. The zygote immediately undergoes meiosis to form four haploid cells called spores. Although these spores are haploid like the “parents,” they contain a new genetic combination from two parents. The spores can remain dormant for various time periods. Eventually, when conditions are favorable, the spores form multicellular haploid structures through many rounds of mitosis (Figure 26.3).
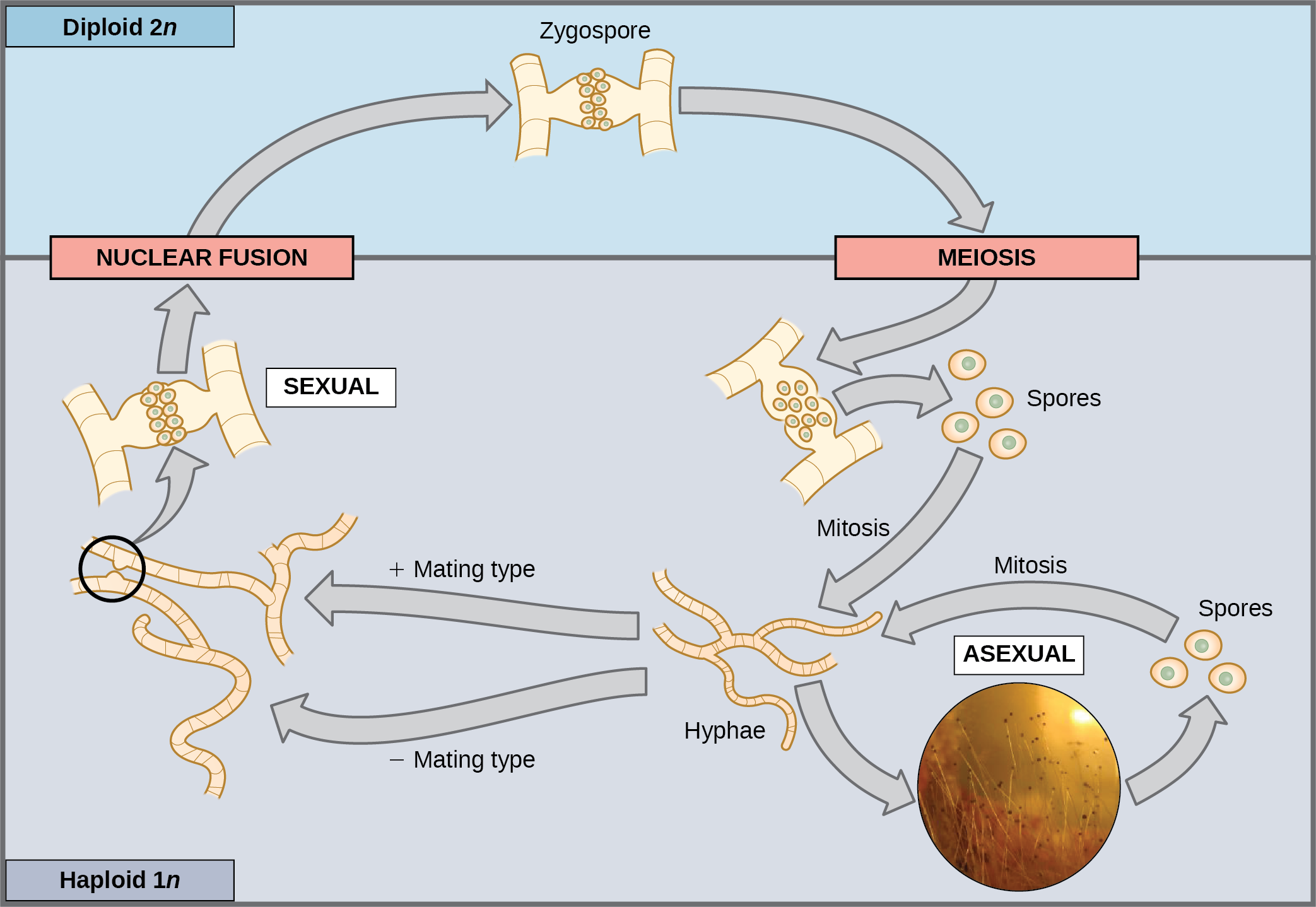
The third life-cycle type, employed by some algae and all plants, is a blend of the haploid-dominant and diploid-dominant extremes. Species with alternation of generations have both haploid and diploid multicellular organisms as part of their life cycle. The haploid multicellular plants are called gametophytes, because they produce gametes from specialized cells. Meiosis is not directly involved in the production of gametes in this case, because the organism that produces the gametes is already haploid. Fertilization between the gametes forms a diploid zygote. The zygote will undergo many rounds of mitosis and give rise to a diploid multicellular plant called a sporophyte. Specialized cells of the sporophyte will undergo meiosis and produce haploid spores. The spores will subsequently develop into the gametophytes (Figure 26.4).
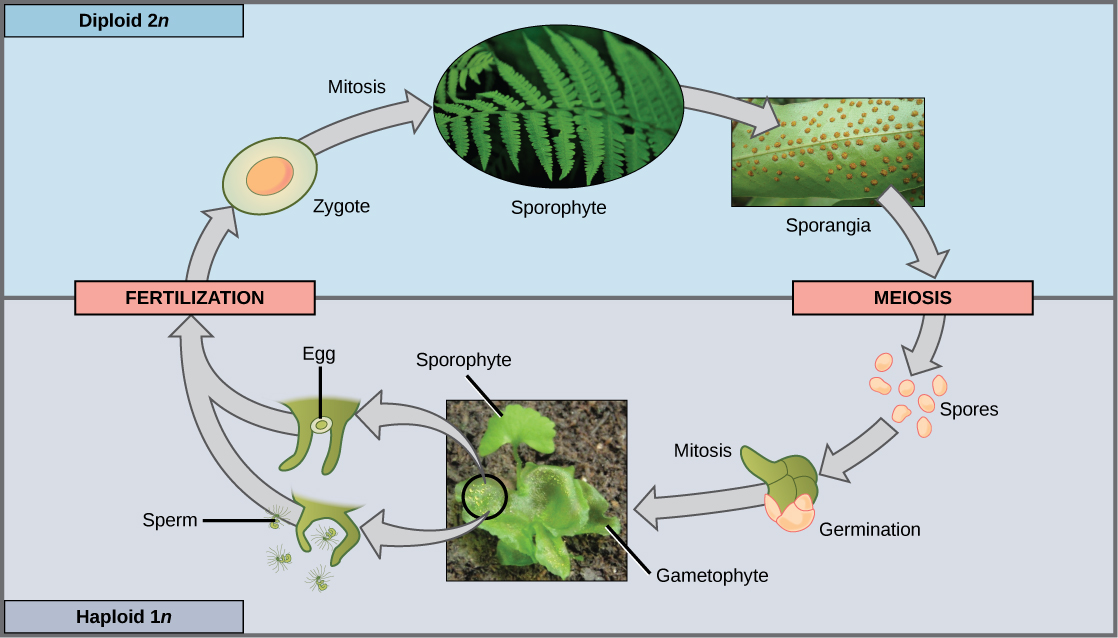
Although all plants utilize some version of the alternation of generations, the relative size of the sporophyte and the gametophyte and the relationship between them vary greatly. In plants such as moss, the gametophyte organism is the free-living plant and the sporophyte is physically dependent on the gametophyte. In other plants, such as ferns, both the gametophyte and sporophyte plants are free-living; however, the sporophyte is much larger. In seed plants, such as magnolia trees and daisies, the gametophyte is composed of only a few cells and, in the case of the female gametophyte, is completely retained within the sporophyte.
Sexual reproduction takes many forms in multicellular organisms. The fact that nearly every multicellular organism on Earth employs sexual reproduction is strong evidence for the benefits of producing offspring with unique gene combinations, though there are other possible benefits as well.
References
Adapted from Clark, M.A., Douglas, M., and Choi, J. (2018). Biology 2e. OpenStax. Retrieved from https://openstax.org/books/biology-2e/pages/1-introduction